COVID-19
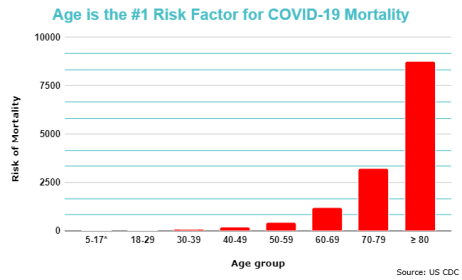
Coronavirus disease 2019 (COVID-19) is an infectious disease caused by the severe acute respiratory syndrome coronavirus 2 (SARS-CoV-2).
The novel coronavirus emerged in late 2019 and rapidly spread throughout the world to become a human pandemic. The substantial suffering and mortality that followed ranks it as one of the greatest pandemics in human history.
The precise molecular reasons for why COVID-19 disproportionately affects the elderly remain unanswered. However, it is apparent is that age is the largest - and by several orders of magnitude - risk factor for mortality.[1]
COVID-19 as a disease of aging
As detailed in the paper, "COVID‐19 is an emergent disease of aging",[2] COVID-19 can be defined as an age-related disease due to the following epidemiological traits:[3][4][5][6]
1) The doubling time for COVID-19 mortality approaches that of the doubling time of 8 years for all-cause mortality/morbidity (as observed for all age-related diseases), per the Gompertz law.
2) There is a greater mortality rate in men, consistent with known sex differences in rates of aging.
3) There is a greater mortality rate for those with age-related comorbidities, consistent with accelerated biological aging.
4) Age dwarfs all other putative risk factors for mortality by several orders of magnitude.
Risk factors are used medicine to assess individual and population risks for certain diseases, in order to guide diagnosis and clinical management. An example of a risk factor is smoking status, which is associated with an approximately 7 fold risk increase of lung cancer.[7]
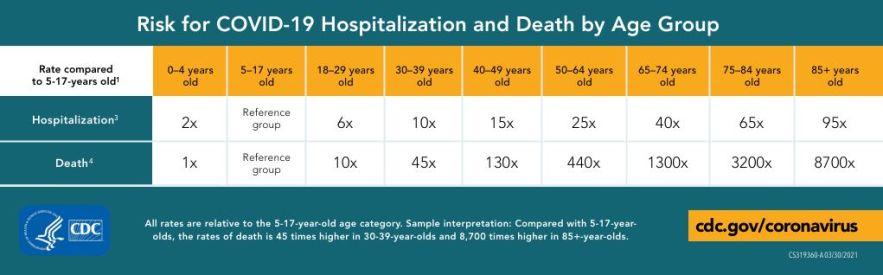
When compared to a reference group of youths aged 5-14, the risk of death for COVID-19 patients over the age of 85 is 8700 times greater, according to data from US States Centers for Disease Control and Prevention (CDC). Other common risk factors identified in COVID-19 scientific discourse are comparatively inconsequential; for example, a history of chronic lung disease confers only a 2 times greater risk of mortality.[8] COVID-19 results in systemic involvement with neurologic, kidney, liver, heart, endocrine, and gut complications;[9] therefore, geroprotectors that treat aging may be valuable for increasing the resilience of multiple aged and vulnerable organ systems.[10][11]
The disproportionate susceptibility to mortality from all causes with age is fundamentally related to age-related decreases in resilience.[12] The multiple systemic consequences of SARS-CoV-2 and the magnitude of risk conferred by age shows that the complex, multisystem phenomenon of biological aging must have a role in disease susceptibility.[2] It has been suggested that an emphasis on the specific organ system of respiration (or the virus itself), rather than the aging host, may be a short-sighted therapeutic strategy.[13] Targeting aging biology mechanisms to improve the resilience of an aging population may also be a strategy to help prevent catastrophic outcomes of future pandemics.[3]
SARS-CoV-2 epidemiology and risk factors
The salient feature of the COVID-19 pandemic was the extreme susceptibility of the elderly to morbidity and mortality.[2] Understanding why and how aging might confer this vulnerability should thus be an important focus of therapeutic strategy.[1][2]
Biomedical scientists and doctors are familiar with the disproportionate susceptibility of older adults to respiratory infections.[1] Similarly to COVID-19, respiratory infections such as pneumonia, influenza and other coronaviruses may show similar mortality risk profiles in the elderly.[2][14]
Influenza (flu) is a notable example of the age-related susceptibility to various pathogen-induced infections, historically linked to annual deaths of 389,000 worldwide, 67% of which occur in people aged 65 or older.[15] Likely reasons for this mortality relationship with age, primarily from the perspective of broad biological mechanisms, include immunosenescence (immune aging) and inflammaging (age-related systemic inflammation).[1][14][16][3]
Aging, comorbidities, and COVID-19
Epidemiological data from several studies, including from the US CDC and the University of Oxford's OpenSAFELY resource, provides limited support for the idea that single comorbidities (typically chronic age-related diseases such as type 2 diabetes or hypertension) are important in isolation as predictors (risk factors) for SARS-CoV-2 mortality.[17][18][19][20] While COVID-19 can be comorbid with another disease, the resulting increase in mortality risk is small; any single comorbidity as a risk factor for mortality remains on the order of single-digit magnitude.[18][19] Data from the CDC in 4,899,447 hospitalized adults show that for patients with greater than 10 comorbidites, the adjusted risk ratio increase for mortality is 3.82 fold (95% CI, 3.45 – 4.23).
Multimorbidity, or having more than two chronic comorbidities, appears to be more important in predicting COVID-19 mortality. In developed countries, the risk of multimorbidity increases exponentially with age, with a prevalence of 20% before age 40, increasing to 75% at age 70.[17][21]
Analyses of the available epidemiological data for COVID-19 reveals that age is the single greatest risk factor for mortality, with no other putative risk factor of comparable magnitude.[1][2] When the presence of comorbidities is controlled for in correlational analyses, age still remains as the dominant risk factor for mortality.[2] This was done by separating apparently healthy older adults without comorbidities from those of similar age with comorbidities.
Similarly, when controlling for age, the predictive value of comorbidities for mortality to SARS-CoV-2 infections fades, indicating substantial confounding by age. Conversely, the residual predictive value of various comorbidities, such as hypertension and cardiovascular diseases, is consistent with the idea that comorbidities may act as partial indicators of biological age, reflecting differences in aging that cannot simply be captured by chronological age.[17] Furthermore, most pre-existing conditions that are risk factors for COVID-19 mortality are age-related diseases, which adds to the idea that biological aging is fundamental to the ability of SARS-CoV-2 (age-dependent mortality) to induce mortality the elderly.[17]
Age and COVID-19 Infection Fatality Ratio (IFR)
In the research field of epidemiology, the infection fatality ratio (IFR) – the ratio of fatalities to total infections – is an important metric for determining the virulence of an infectious disease.[22] In the early stages of the pandemic, determining the IFR of COVID-19 was subject to significant uncertainty due to lack of data.
One estimate found that the IFR of a given country's population was significantly dependent on the age structure, indicating that IFR is age-specific.[23] For example, Japan had the highest estimated IFR of 1.09% (95% credible interval, 0.94–1.26%), whereas Kenya had the lowest estimated IFR of 0.09% (95% credible interval, 0.08–0.10%).[23] This predominantly reflects demographic differences, partly related to a significantly larger proportion of elderly citizens in Japan .
From the perspective of COVID-19 mortality, some countries with minimal public health resources have fared better than expected during the SARS-CoV-2 pandemic. Instead, the acute impacts of COVID-19 felt by such countries are anticipated to be related to secondary or indirect effects.[24] In developing countries that have a younger demographic structure, age-specific IFRs may require consideration by public health stakeholders. Neglecting to consider tradeoffs that apply to populations with differing susceptibility to diseases may cause unnecessary harm. Some have advocated for vaccination strategies with a major focus on age.[25]
Secondary effects of policy decisions may also affect competing causes of mortality, which will vary depending on population and disease demographics, as well as socioeconomic factors. For example, infectious disease experts estimate that disruptions to malaria vaccinations in Africa due to the pandemic could double Malaria mortality in 2020.[24][26]
Biological aspects linking COVID-19 to aging
SARS-CoV-2 causes senescence in human cells
The accumulation of senescent cells with aging drives the secretion of a host of pro-inflammatory factors, known as the senescence-associated secretory phenotype (SASP).[27] This has been linked to various chronic diseases of aging, such as Alzheimer's, atherosclerosis, and various cancers.[28] It is well known that many viral and bacterial infections can induce cellular senescence, which accounts for some of the detrimental effects of infection.[29][30][31][32]
Cellular senescence is a major biological mechanism linked to aging which has been hypothesized to contribute to poor COVID-19 outcomes. It may help explain pathophysiological features such as macrophage dysfunction and the cytokine storm, as well as clinical manifestations such as the disproportionate mortality and reduced vaccine response of the biologically old.[32] There is considerable overlap between ailments that are driven by senescence and that of COVID-19, such as lung dysfunction, frailty, cognitive decline, and heart damage.[33] Inflammatory factors that make up the SASP, such as IL-6 and IL-8, have been associated with poor outcomes in COVID-19.[34]
A recent study showed that in non-senescent cells SARS-CoV-2 is able to cause cellular senescence, while in senescent cells, increases the expression of the pro-inflammatory SASP that is associated with age-related diseases.[33] The authors also found an increased expression of the senescent cell marker p16INK4a in lungs of patients who died to acute COVID-19, compared to dead controls who did not have diseases related to the lungs. Consistent with the in vitro data, this suggests that SARS-CoV-2 induces senescence.[33]
In an in vitro model, senescent human kidney cells exposed to the SARS-CoV-2 spike protein-1 show an increase in senescence-associated inflammatory factors.[35] Additionally, human lung epithelial cells exposed to various senescent cells show a significantly greater expression of ACE2 and TMPRSS2, which are COVID-19 viral entry genes.[35] Similarly, another study of COVID-19 infected patients found evidence of cell senescence in airway mucosa, and higher expression of SASP inflammatory factors in blood.[36]
Senolytic drugs selectively remove senescent cells from the body, which have been shown to delay and even partly reverse multiple age-related diseases, resulting in expanded healthy lifespan in mice.[37][38] In a preclinical study in old mice, the senolytic drug fisetin halved mortality due to mouse hepatitis virus (MHV), a β-coronavirus in the same family as SARS-CoV-2, with reduced expression of SASP inflammatory markers and improved immune function.[35] This mouse study also showed similar therapeutic benefit with dasatinib plus quercetin (D+Q), which is another class of senolytics used in combination.[35] Consistent with COVID-19 epidemiology, young but not old mice were resistant to MHV infection; benefits of senolytics use were specific to the old mice.[35]
Another study investigating senolysis in golden hamsters and mice infected with SARS-CoV-2 found significantly increased survival following treatment with senolytic drugs.[36] In hamsters, the combination of dasatinib and quercetin led to a substantial reduction in histopathological features of lung disease with SASP factor expression more closely mirroring that of healthy control hamsters. In small groups of 5 mice, both D+Q and fisetin each had 5 of 5 mice survive at day 6, compared to 3 of 5 in the control group. The senolytic navitoclax did not exhibit as consistent an effect on several measures in either animal models.[36]
COVID-19 may accelerate aging in humans
Long COVID, also known as Post COVID, Long-Hauler's Syndrome or post-acute sequelae of COVID-19 (PASC), describes a non-specific cluster of long-term health consequences affecting multiple organ systems in those previously infected by COVID-19. This generally refers to chronic, residual consequences that range from neuropsychiatric ailments, to metabolic disease, and even frailty.
One study of nursing home residents found an association between COVID-19 infection and exhibiting a state similar to accelerated aging.[39] This included greater declines in walking speed and handgrip strength, with worsening of frailty as measured via a nursing-home specific frailty index.[39] Due to preclinical data showing that SARS-CoV-2 can drive cellular senescence in human cells, an observational study is ongoing to determine whether senescence markers are increased in long COVID patients versus uninfected controls (Cellular Senescence and COVID-19 Long-Hauler Syndrome; NCT04903132).[33] Clinical data is generally lacking to provide strong evidence of accelerated aging due to COVID-19.
Currently there is a lack of research for Long COVID, but it is speculated that the biology of aging is closely related, in part due to multimorbidity from various affected organs.[40] With the known ability of SARS-CoV-2 to induce cellular senescence in mice in vivo and human cells in vitro, and the clinical characteristics of COVID-19, it has been hypothesized that the virus may accelerate aging in infected patients.[33][40]
Immune aging and vulnerability to infectious diseases
Both immunosenescence and inflammaging are well-known biological mechanisms that underlie age-related susceptibility to various infectious diseases, such as the common cold, influenza, and pneumonia.
Immunosenescence is characterized by the age-related decline in the host's ability to mount an appropriate immune response to an infectious agent.[41] A major reason for this decline is related to atrophy of the thymus (thymic involution), an important organ that is important for generating naive T cells. It is therefore central to the adaptive immune system, which is integral to providing long-term protection against old and new infectious pathogens.
Inflammaging describes the age-related systemic inflammation, which affects various systems across the body that leads to organ and tissue dysfunction.[41] It is known that with aging, dysregulation of the innate immune system results in chronic hyperactivation, and becomes a key driver of multiple age-related diseases via chronic inflammation.[42]
Human clinical trials targeting the biology of aging for COVID-19
As published in various journals, including The Lancet Healthy Longevity, various professors, scientists, and physicians have advocated the need for clinical trials targeting the biology of aging for COVID-19.[3][2][43] Investigating drugs that target aging biology could be an important strategy to improving immune function in the elderly,[44] because severe outcomes and mortality are linked primarily to aging (i.e. immunosenescence and inflammaging). Because of the far reaching consequences of biological aging, this has implications for not just seasonal infectious diseases such as influenza, but also for pandemics such as COVID-19, as well as other diseases that disproportionately affect the elderly.[45]
The following drugs are currently being investigated in clinical trials for the treatment of COVID-19. Investigational are not currently regarded as safe or effective for this indication.
RTB101
RTB101 (dactolisib) is an FDA-approved drug used in oncology, with primary mechanism of action of ATP-competitive PI3K/mTOR dual kinase inhibition.
Inhibition of mTOR has been shown in human clinical studies to improve immune function in older adults.[46][47] Phase 2 studies have shown that mTOR inhibitors can reduce viral infection severity and improve the effectiveness of vaccination, such as for influenza. This is in contrast to the known ability for rapamycin (and its analogues, e.g. everolimus) to induce immunosuppression, which is related to its primary indication as an FDA-approved liver and lung transplant drug.
Following the success of two phase 2 clinical trials investigating mTOR inhibition for targeting the aging immune system, RTB101 (dactolisib) is currently being pursued for the treatment of COVID-19 in a phase 2a placebo-controlled trial, exploring the potential for preventing severe disease in asymptomatic elderly adults who have been exposed to COVID-19.[48][49]
This trial is being run by the biopharmaceutical company resTORbio, with funding from the National Institute on Aging.
The primary rationale for why RTB101 has potential to show benefit for the 2019 strain of coronavirus stems from phase 2b and phase 3 clinical trial data of RTB101, showing fewer laboratory-confirmed (non-COVID-19) coronavirus cases, and reduced severity of coronavirus infection in elderly adults aged ≥ 65.[50] The phase 3 randomized, double-blind, placebo-controlled clinical trial for RTB101 was withdrawn in November 2019, following failure to meet its primary endpoint of reducing symptoms of respiratory tract infections (RTIs) in adults aged ≥ 65, with or without laboratory-confirmed viral illness.[50]
Considering the positive clinical data from the phase 2 trials, which involved investigations of both everolimus (a rapalog) and RTB101, the failure of the later phase 3 trial has been attributed to the decision to move ahead with only RTB101.[51] This is because only rapamycin, an allosteric inhibitor of mTOR, has demonstrated the extension of healthspan and lifespan in various animal models, with a rejuvenation of immune function; while RTB101, an ATP-competitive PI3K/mTOR dual kinase inhibitor of differing mechanism of action to rapamycin, has not yet demonstrated these effects in animal models.[51] The decision to omit the use of everolimus and continue with RTB101 in the phase 3 trial is speculated to be related to the off-patent status; it would be prohibitive for a publicly-traded company with fiduciary responsibility to shareholders to advance a drug with no commercial potential into late-stage clinical trials.[51]
Joan Mannick, the Chief Medical Officer of resTORbio, has since justified the trial's failure at several medical conferences with the belief that it was related to a change in the primary endpoint. This was requested by the US FDA when the trial advanced from phase 2 to 3. In the prior successful phase 2 trial, the primary endpoint had been RTI symptoms with laboratory confirmation of a causative virus, but this endpoint was changed to RTI symptoms with or without laboratory-confirmed viral illness in the failed phase 3 trial.[50] This is a problem for interpreting the clinical trial because it was not the original endpoint for which the drug had showed benefit in. Mannick has also argued that this introduced more noise to the trial, as older adults inherently experience respiratory tract symptoms at greater rates, even without laboratory-confirmation of viral presence.
COVID-19
Unpublished data from the phase 2 trial of RTB101 for COVID-19 among nursing home patients treated within 3 days from testing positive saw promising results.[52] None of those treated with RTB101 developed symptoms (n=18), while the placebo treated control group had 4 severe cases of disease and 2 deaths. While this was a statistically significant finding, larger trials are warranted for further evidence of potential benefit.[52]
Fisetin
Fisetin is a senolytic that is being investigated in two phase 2 clinical trials at the Mayo Clinic,[53] with the second trial being funded by the National Institute on Aging of the US NIH.[54]
For SARS-CoV-2, fibrosis of the lung tissue is a well-known serious complication following acute respiratory distress syndrome (ARDS). Senolytic drugs are capable of slowing and/or reversing age-related lung fibrosis in mice, as well as promoting health in multiple tissues/organs, and so may provide benefit for both acute and chronic manifestations of COVID-19.[55] The pro-inflammatory secretions of senescent cells have been identified as key drivers of tissue and organ dysfunction with aging, which have been linked to the cytokine storm associated with COVID-19 ARDS.[55] It is hypothesized that senolytics may alleviate the age-associated decline in lung function, in addition to improving immune function such as via the clearance of senescent T cells.[55][56]
BGE-175
BGE-175 is an investigational new drug for COVID-19 that was previously studied in phase 1 to 3 trials for allergic rhinitis involving 2400 study participants. It is an oral inhibitor of prostaglandin D2 (PGD2) DP1 signaling.[57] It is being advanced in clinical trials by BioAge, a privately-held biotechnology company that aims to treat aging and aging-related diseases.
While BGE-175 has not been shown to extend healthspan and/or lifespan in published animal models, the company claims that the rationale for its consideration as an aging drug is based on targeting the apelin pathway that is associated with healthspan and lifespan in humans.[58][59]
- ↑ Jump up to: 1.0 1.1 1.2 1.3 1.4 Mueller, A. L., McNamara, M. S., & Sinclair, D. A. (2020). Why does COVID-19 disproportionately affect older people?. Aging (Albany NY), 12(10), 9959.
- ↑ Jump up to: 2.0 2.1 2.2 2.3 2.4 2.5 2.6 2.7 Santesmasses, D., Castro, J. P., Zenin, A. A., Shindyapina, A. V., Gerashchenko, M. V., Zhang, B., ... & Gladyshev, V. N. (2020). COVID‐19 is an emergent disease of aging. Aging cell, 19(10), e13230. https://onlinelibrary.wiley.com/doi/10.1111/acel.13230
- ↑ Jump up to: 3.0 3.1 3.2 3.3 Barzilai, N., Appleby, J. C., Austad, S. N., Cuervo, A. M., Kaeberlein, M., Gonzalez-Billault, C., ... & Sierra, F. (2020). Geroscience in the Age of COVID-19. Aging and disease, 11(4), 725.
- ↑ Promislow, D. E. (2020). A geroscience perspective on COVID-19 mortality. The Journals of Gerontology: Series A, 75(9), e30-e33.
- ↑ Alberts, S. C., Archie, E. A., Gesquiere, L. R., Altmann, J., Vaupel, J. W., & Christensen, K. (2014). The male-female health-survival paradox: a comparative perspective on sex differences in aging and mortality. In Sociality, hierarchy, health: comparative biodemography: a collection of papers. National Academies Press (US).
- ↑ Sierra, F. (2020). Geroscience and the Coronavirus Pandemic: The Whack‐a‐Mole Approach is not Enough. Journal of the American Geriatrics Society, 68(5), 951.
- ↑ O’Keeffe, L. M., Taylor, G., Huxley, R. R., Mitchell, P., Woodward, M., & Peters, S. A. (2018). Smoking as a risk factor for lung cancer in women and men: a systematic review and meta-analysis. BMJ open, 8(10), e021611.
- ↑ Williamson, E. J., Walker, A. J., Bhaskaran, K., Bacon, S., Bates, C., Morton, C. E., ... & Goldacre, B. (2020). Factors associated with COVID-19-related death using OpenSAFELY. Nature, 584(7821), 430-436.
- ↑ Gupta, A., Madhavan, M. V., Sehgal, K., Nair, N., Mahajan, S., Sehrawat, T. S., ... & Landry, D. W. (2020). Extrapulmonary manifestations of COVID-19. Nature medicine, 26(7), 1017-1032.
- ↑ Sierra, F. (2020). Geroscience and the Coronavirus Pandemic: The Whack‐a‐Mole Approach is not Enough. Journal of the American Geriatrics Society, 68(5), 951.
- ↑ Barzilai, N., Appleby, J. C., Austad, S. N., Cuervo, A. M., Kaeberlein, M., Gonzalez-Billault, C., ... & Sierra, F. (2020). Geroscience in the Age of COVID-19. Aging and disease, 11(4), 725.
- ↑ Pyrkov, T. V., Avchaciov, K., Tarkhov, A. E., Menshikov, L. I., Gudkov, A. V., & Fedichev, P. O. (2021). Longitudinal analysis of blood markers reveals progressive loss of resilience and predicts human lifespan limit. Nature Communications, 12(1), 1-10. Chicago
- ↑ Sierra, F. (2020). Geroscience and the Coronavirus Pandemic: The Whack‐a‐Mole Approach is not Enough. Journal of the American Geriatrics Society, 68(5), 951.
- ↑ Jump up to: 14.0 14.1 Yoshikawa, T. T. (2000). Epidemiology and unique aspects of aging and infectious diseases. Clinical Infectious Diseases, 30(6), 931-933.
- ↑ Paget, J., Spreeuwenberg, P., Charu, V., Taylor, R. J., Iuliano, A. D., Bresee, J., ... & Viboud, C. (2019). Global mortality associated with seasonal influenza epidemics: New burden estimates and predictors from the GLaMOR Project. Journal of global health, 9(2).
- ↑ Akbar, A. N., & Gilroy, D. W. (2020). Aging immunity may exacerbate COVID-19. Science, 369(6501), 256-257.
- ↑ Jump up to: 17.0 17.1 17.2 17.3 Polidori, M. C., Sies, H., Ferrucci, L., & Benzing, T. (2021). COVID-19 mortality as a fingerprint of biological age. Ageing Research Reviews, 101308.
- ↑ Jump up to: 18.0 18.1 Williamson, E. J., Walker, A. J., Bhaskaran, K., Bacon, S., Bates, C., Morton, C. E., ... & Goldacre, B. (2020). Factors associated with COVID-19-related death using OpenSAFELY. Nature, 584(7821), 430-436.
- ↑ Jump up to: 19.0 19.1 Kompaniyets, L., Pennington, A. F., Goodman, A. B., Rosenblum, H. G., Belay, B., Ko, J. Y., ... & Gundlapalli, A. V. (2021). Peer Reviewed: Underlying Medical Conditions and Severe Illness Among 540,667 Adults Hospitalized With COVID-19, March 2020–March 2021. Preventing Chronic Disease, 18.
- ↑ Goodman, K. E., Magder, L. S., Baghdadi, J. D., Pineles, L., Levine, A. R., Perencevich, E. N., & Harris, A. D. (2020). Impact of Sex and Metabolic Comorbidities on Coronavirus Disease 2019 (COVID-19) Mortality Risk Across Age Groups: 66 646 Inpatients Across 613 US Hospitals. Clinical Infectious Diseases.
- ↑ Violan, C., Foguet-Boreu, Q., Flores-Mateo, G., Salisbury, C., Blom, J., Freitag, M., ... & Valderas, J. M. (2014). Prevalence, determinants and patterns of multimorbidity in primary care: a systematic review of observational studies. PloS one, 9(7), e102149.
- ↑ Levin, A. T., Hanage, W. P., Owusu-Boaitey, N., Cochran, K. B., Walsh, S. P., & Meyerowitz-Katz, G. (2020). Assessing the age specificity of infection fatality rates for COVID-19: systematic review, meta-analysis, and public policy implications. European journal of epidemiology, 1-16.
- ↑ Jump up to: 23.0 23.1 O’Driscoll, M., Dos Santos, G. R., Wang, L., Cummings, D. A., Azman, A. S., Paireau, J., ... & Salje, H. (2021). Age-specific mortality and immunity patterns of SARS-CoV-2. Nature, 590(7844), 140-145.
- ↑ Jump up to: 24.0 24.1 Weiss, D. J., Bertozzi-Villa, A., Rumisha, S. F., Amratia, P., Arambepola, R., Battle, K. E., ... & Gething, P. W. (2021). Indirect effects of the COVID-19 pandemic on malaria intervention coverage, morbidity, and mortality in Africa: a geospatial modelling analysis. The Lancet Infectious Diseases, 21(1), 59-69.
- ↑ Privor-Dumm, L. A., Poland, G. A., Barratt, J., Durrheim, D. N., Knoll, M. D., Vasudevan, P., ... & Bonanni, P. (2021). A global agenda for older adult immunization in the COVID-19 era: a roadmap for action. Vaccine, 39(37), 5240-5250.
- ↑ Sherrard-Smith, E., Hogan, A. B., Hamlet, A., Watson, O. J., Whittaker, C., Winskill, P., ... & Churcher, T. S. (2020). The potential public health consequences of COVID-19 on malaria in Africa. Nature medicine, 26(9), 1411-1416.
- ↑ Campisi, J., & Di Fagagna, F. D. A. (2007). Cellular senescence: when bad things happen to good cells. Nature reviews Molecular cell biology, 8(9), 729-740.
- ↑ He, S., & Sharpless, N. E. (2017). Senescence in health and disease. Cell, 169(6), 1000-1011.
- ↑ Wei, W., & Ji, S. (2018). Cellular senescence: molecular mechanisms and pathogenicity. Journal of cellular physiology, 233(12), 9121-9135.
- ↑ Chuprin, A., Gal, H., Biron-Shental, T., Biran, A., Amiel, A., Rozenblatt, S., & Krizhanovsky, V. (2013). Cell fusion induced by ERVWE1 or measles virus causes cellular senescence. Genes & development, 27(21), 2356-2366.
- ↑ Martínez, I., García-Carpizo, V., Guijarro, T., Garcia-Gomez, A., Navarro, D., Aranda, A., & Zambrano, A. (2016). Induction of DNA double-strand breaks and cellular senescence by human respiratory syncytial virus. Virulence, 7(4), 427-442.
- ↑ Jump up to: 32.0 32.1 Kelley, W. J., Zemans, R. L., & Goldstein, D. R. (2020). Cellular senescence: friend or foe to respiratory viral infections?. European Respiratory Journal, 56(6).
- ↑ Jump up to: 33.0 33.1 33.2 33.3 33.4 Tripathi, U., Nchioua, R., Prata, L. G. L., Zhu, Y., Gerdes, E. O. W., Giorgadze, N., ... & Kirkland, J. L. (2021). SARS-CoV-2 causes senescence in human cells and exacerbates the senescence-associated secretory phenotype through TLR-3. Aging (Albany NY), 13(18), 21838.
- ↑ Laing, A. G., Lorenc, A., Del Barrio, I. D. M., Das, A., Fish, M., Monin, L., ... & Hayday, A. C. (2020). A dynamic COVID-19 immune signature includes associations with poor prognosis. Nature medicine, 26(10), 1623-1635.
- ↑ Jump up to: 35.0 35.1 35.2 35.3 35.4 Camell, C. D., Yousefzadeh, M. J., Zhu, Y., Prata, L. G. L., Huggins, M. A., Pierson, M., ... & Robbins, P. D. (2021). Senolytics reduce coronavirus-related mortality in old mice. Science.
- ↑ Jump up to: 36.0 36.1 36.2 Lee, S., Yu, Y., Trimpert, J., Benthani, F., Mairhofer, M., Richter-Pechanska, P., ... & Schmitt, C. A. (2021). Virus-induced senescence is a driver and therapeutic target in COVID-19. Nature, 1-7.
- ↑ Yousefzadeh, M. J., Zhu, Y. I., McGowan, S. J., Angelini, L., Fuhrmann-Stroissnigg, H., Xu, M., ... & Niedernhofer, L. J. (2018). Fisetin is a senotherapeutic that extends health and lifespan. EBioMedicine, 36, 18-28.
- ↑ Baker, D. J., Wijshake, T., Tchkonia, T., LeBrasseur, N. K., Childs, B. G., Van De Sluis, B., ... & Van Deursen, J. M. (2011). Clearance of p16 Ink4a-positive senescent cells delays ageing-associated disorders. Nature, 479(7372), 232-236.
- ↑ Jump up to: 39.0 39.1 Greco, G. I., Noale, M., Trevisan, C., Zatti, G., Dalla Pozza, M., Lazzarin, M., ... & Sergi, G. (2021). Increase in Frailty in Nursing Home Survivors of Coronavirus Disease 2019: Comparison With Noninfected Residents. Journal of the American Medical Directors Association, 22(5), 943-947.
- ↑ Jump up to: 40.0 40.1 Gerdes, E. O. W., Vanichkachorn, G., Verdoorn, B. P., Hanson, G. J., Joshi, A. Y., Murad, M. H., Rizza, S. A., Tchkonia, T., & Kirkland, J. L. (2021). Role of senescence in the chronic health consequences of COVID-19. Translational Research.
- ↑ Jump up to: 41.0 41.1 Furman, D., Campisi, J., Verdin, E., Carrera-Bastos, P., Targ, S., Franceschi, C., ... & Slavich, G. M. (2019). Chronic inflammation in the etiology of disease across the life span. Nature medicine, 25(12), 1822-1832.
- ↑ Shaw, A. C., Goldstein, D. R., & Montgomery, R. R. (2013). Age-dependent dysregulation of innate immunity. Nature Reviews Immunology, 13(12), 875-887.
- ↑ Mannick, J. B., Teo, G., Bernardo, P., Quinn, D., Russell, K., Klickstein, L., ... & Shergill, S. (2021). Targeting the biology of ageing with mTOR inhibitors to improve immune function in older adults: phase 2b and phase 3 randomised trials. The Lancet Healthy Longevity, 2(5), e250-e262.
- ↑ Cox, L. S., Bellantuono, I., Lord, J. M., Sapey, E., Mannick, J. B., Partridge, L., ... & Witham, M. D. (2020). Tackling Immunosenescence to improve COVID-19 outcomes and vaccine response in older adults. The Lancet Healthy Longevity, 1(2), e55-e57.
- ↑ Akbar, A. N., & Gilroy, D. W. (2020). Aging immunity may exacerbate COVID-19. Science, 369(6501), 256-257.
- ↑ Mannick, J. B., Del Giudice, G., Lattanzi, M., Valiante, N. M., Praestgaard, J., Huang, B., ... & Klickstein, L. B. (2014). mTOR inhibition improves immune function in the elderly. Science translational medicine, 6(268), 268ra179-268ra179.
- ↑ Mannick, J. B., Morris, M., Hockey, H. U. P., Roma, G., Beibel, M., Kulmatycki, K., ... & Klickstein, L. B. (2018). TORC1 inhibition enhances immune function and reduces infections in the elderly. Science translational medicine, 10(449).
- ↑ Restorbio Inc. A Randomized, Double-Blind, Placebo-Controlled Phase 2a Study of RTB101 as COVID-19 Post-Exposure Prophylaxis in Adults Age ≥65 Years. Clinical trial registration, NCT04584710, clinicaltrials.gov, 5 Feb. 2021. clinicaltrials.gov, https://clinicaltrials.gov/ct2/show/NCT04584710.
- ↑ Restorbio Inc. Randomized Double Blind Placebo-Controlled Study to Determine If Prophylaxis With RTB101 Compared to Placebo Reduces Severity of Lab Confirmed COVID19 in Adults ≥65 Years in a Nursing Home in Which ≥1 Person(s) Have Lab Confirmed COVID19. Clinical trial registration, NCT04409327, clinicaltrials.gov, 5 Feb. 2021. clinicaltrials.gov, https://clinicaltrials.gov/ct2/show/NCT04409327.
- ↑ Jump up to: 50.0 50.1 50.2 Mannick, J. B., Teo, G., Bernardo, P., Quinn, D., Russell, K., Klickstein, L., ... & Shergill, S. (2021). Targeting the biology of ageing with mTOR inhibitors to improve immune function in older adults: phase 2b and phase 3 randomised trials. The Lancet Healthy Longevity, 2(5), e250-e262.
- ↑ Jump up to: 51.0 51.1 51.2 Kaeberlein, M. (2020). RTB101 and immune function in the elderly: Interpreting an unsuccessful clinical trial. Translational Medicine of Aging, 4, 32-34.
- ↑ Jump up to: 52.0 52.1 Metabesity Conference, 2021, 'Targeting Metabesity 2021: Evidence 3 – Establishing Clear Pathways for Healthspan Products' https://www.youtube.com/watch?v=mg4jL_WdFd0
- ↑ Kirkland, James L. COVID-FISETIN: A Phase 2 Placebo-Controlled Pilot Study in SARS-CoV-2 of Fisetin to Alleviate Dysfunction and Excessive Inflammatory Response in Hospitalized Adults. Clinical trial registration, NCT04476953, clinicaltrials.gov, 16 Feb. 2021. clinicaltrials.gov, https://clinicaltrials.gov/ct2/show/NCT04476953.
- ↑ PhD, James L. Kirkland, MD. COVID-FIS: A Phase 2 Placebo-Controlled Pilot Study in COVID-19 of Fisetin to Alleviate Dysfunction and Excessive Inflammatory Response in Older Adults in Nursing Homes. Clinical trial registration, NCT04537299, clinicaltrials.gov, 1 June 2021. clinicaltrials.gov, https://clinicaltrials.gov/ct2/show/NCT04537299.
- ↑ Jump up to: 55.0 55.1 55.2 Nehme, J., Borghesan, M., Mackedenski, S., Bird, T. G., & Demaria, M. (2020). Cellular senescence as a potential mediator of COVID‐19 severity in the elderly. Aging Cell, 19(10), e13237
- ↑ Akbar, A. N., & Gilroy, D. W. (2020). Aging immunity may exacerbate COVID-19. Science, 369(6501), 256-257.
- ↑ https://www.businesswire.com/news/home/20210322005146/en/BioAge-Initiates-Phase-2-Trial-of-BGE-175-to-Treat-COVID-19-by-Reversing-Immune-Aging-a-Key-Cause-of-Morbidity-and-Mortality-in-Older-Patients
- ↑ https://www.fiercebiotech.com/biotech/bioage-picks-up-muscle-aging-program-from-amgen-bringing-pipeline-count-to-3
- ↑ Zhou, Q., Chen, L., Tang, M., Guo, Y., & Li, L. (2018). Apelin/APJ system: A novel promising target for anti-aging intervention. Clinica Chimica Acta, 487, 233-240.