Senolytics
Senolytics (from senile - decrepit and lytic - lysing, destroying) - a class of drugs thought to target aging, a distinctive feature of which is the ability to selectively initiate the death of 'aged' cells[1][2]
Prehistory
The appearance of senolytics was foreseen in the 19th century in studies of the effect of highly dilute solutions of hydrogen cyanide, called prussic acid, on cell survival. It was found that unlike young cells, old and cancerous cells quickly die from such exposure.[3] These data were used to scientifically explain a paradox known since ancient times as mithridatism and later called hormesis: taking very small doses of a non-cumulative poison sometimes leads to better health.[4] Obviously, toxins such as hydrogen cyanide, by means of mitohormesis (due to mitophagy), remove old cells with defective mitochondria unable to withstand temporary hypoxia.[5]
In 1837, the German scientists von Liebig and Woehier found that hydrogen cyanide can be obtained from the constituent of apricot seeds and bitter almonds the cyanogenic glycoside amygdalin. Its simpler derivative obtained by amygdalin hydrolysis referred to as laetrile (patented 1961) or vitamin B17, although it is not classified as a vitamin, are still sold as dietary supplements. It was discovered that low doses of amygdalin may exhibit protective effects, yet higher amygdalin concentrations may be toxic to the biological system.[6][7] Rumors about the healthy aging effect of amygdalin were added to by stories about centenarians among the Hunza people who use apricot seeds as food.[8]
A principle of synergistic synthetic lethality was developed to search for drugs that have a detrimental effect on the cell only when they are combined.[9] “Synthetic lethality” is defined as a type of interaction in which the combination of harmful to the cell influence results in cell death. Synthetic lethality is thought to kill cancer or senescent cells specifically without affecting normal cells by acting on specific genes or common molecular pathways regulated in the aging or carcinogenesis process. [10]
Senescent cells as a factor of aging and age-associated diseases
The progressive and gradual decline of an aging body is one of the main causes or predisposing factors to developing aging-related diseases, such as CVD (cardiovascular disease), cancer, diabetes, and kidney disease, ultimately leading to death.
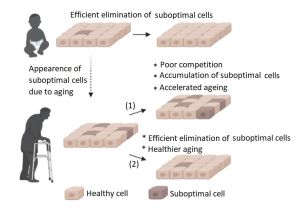
One key factor causing the decline of tissue homeostasis, systemic inflammation, DNA damage etc. that contribute to disease are the so-called senescent cells that are known to accumulate with aging.[12][13][14]Cellular Senescence is a form of durable cell cycle arrest elicited in response to a wide range of stimuli. Senescent cells are sometimes referred to as "old" or "zombie" cells are cells that have stopped dividing and growing but remain metabolically active.[15][16]
Three characteristics thought to define senescent cells are irreversible cell cycle arrest, the secretion of pro-inflammatory senescence-associated secretory phenotype (SASP), and resistance to apoptosis. However, it has become increasingly appreciated that there senescent cells are difficult to define, as benefits or detriments to health depend on the context, e.g. being tissue or organ-specific[17][18].
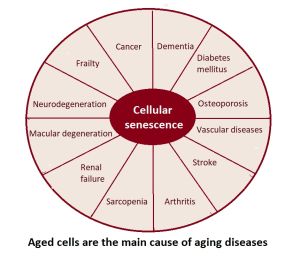
Senescence is often viewed as a double-edged sword with both beneficial and detrimental effects.[20][18][21]
Among its beneficial actions, senescence was shown to promote wound repair, developmental morphogenesis, and tumor suppression, mainly by triggering cell cycle arrest and the release of specific cytokines necessary for wound healing.[22][23] Senescent cells can contribute to tissue repair by secreting growth factors that promote the proliferation and differentiation of nearby stem cells. This process is important for the healing of injuries and the maintenance of tissue and organ function. A study of salamander limb regeneration found that implanted senescent cells, prior to promote cell proliferation, enhance muscle dedifferentiation, a critical process underlying successful limb regeneration, and that senescent cells are able to modulate this muscle dedifferentiation directly, through the secretion of paracrine factors including WNT and FGF ligands.[24] Senescent cells can play a role in the body's response to stress, including tissue damage and oxidative stress. When cells experience stress or DNA damage, they may enter a state of senescence to prevent further division and growth, which can help to limit the spread of damaged or potentially cancerous cells. In this way, senescence can act as a barrier to the development of cancer.
Although senescent cells can play a role in the body's response to stress and tissue repair, their accumulation over time is thought to contribute to the aging process and the development of age-related diseases.[25] Among its detrimental actions, senescent cells, even though their abundance in aging or diseased tissues is very low,[26] contribute to chronic inflammation and tissue degeneration mainly derived from the production of the pro-inflammatory cytokines, growth factors, and extracellular matrix proteases that comprise their secretion - SASP (senescence associated secretory phenotype), which can contribute to tissue damage, inflammation, and the progression of age-related diseases.[19] In this regard, the SASP was shown to alter tissue function and to accelerate the aging process by recruiting immune cells and extracellular matrix-remodeling complexes. Accordingly, in young individuals, senescence plays a key role in tumor surveillance and tissue repair, whereas in older individuals, the accumulation of senescent cells has been associated with tissue dysfunction and chronic conditions like cancer, cardiovascular disease and neurodegeneration.[19] Importantly, clearance of senescent cells using genetic approaches or senolytic drugs has been shown to improve tissue function in different in vivo models of aging and age-associated diseases.[19] In addition, senescent cells can also promote the development of cancer by evading cell death and contributing to the accumulation of genetic mutations.[27] They can also impair the function of nearby healthy cells, leading to a decline in tissue and organ function - a phenomenon known as paracrine senescence, where secreted senescence factors and extracellular vesicles (EVs)[28] can induce senescence (secondary due to paracrine senescence niche) of neighboring cells.[29][30]
Multicellular organisms usually contain tissue-resident stem and progenitor cells that consistently give rise to new cells for tissue building and regeneration.[31] However, in order for new cells to take their place, it is necessary to first remove the old ones that have lost their effectiveness. While the body is young, it easily removes senescent cells with the help of the immune system[15][32][33] and by selecting the fittest cells with the help of Cell Competition,[34][11][35][36] maintaining tissue and organ health.[37]
Markers of cellular senescence
The negative impact of SASP components on the body can be weakened by removing aged cells. There is no single biomarker present in all senescent cells, and conversely the presence of a single biomarker is not a hard indication that a cell is senescent. Therefore identification of senescent cells generally involves multiple biomarkers, of which senescence-associated pH6 β-galactosidase,[38] p21CIP1/WAF1,[39] p16INK4a, and intracellular lipofuscin accumulation[40] are prominent.[41] One of the signs of a cell switching to the path of irreversible aging is the de-repression of the p16INK4a gene, which maintains the viability of senescent cells by preventing their apoptosis.[42] It has been shown that the removal of senescent p16INK4a-positive cells can slow the progression of age-related disorders even at later stages of life.[43][44] However, whether cells that express p16INK4a are actually 'senescent cells', and if removal of such cells could cause harm in specific contexts has been questioned by more recent work.[18] Moreover, a limitation of this approach and similar methods that use genetic engineering[45] is the need for manipulations of the genome. It can instead be easier to use small molecule senolytics capable of activating the process of selective destruction of aged cells.
By removing aged cells, senolytics are thought to start the “on demand” regeneration process, the purpose of which is to fill the formed space with new cells, such as by differentiation of resident stem cells.[46] Notably, this is dependent on the availability of stem cell pools which are known to decline with aging, and this has been identified as a theoretical limitation of senolytics, if the lack of such stem cells means new tissue is not formed. It has also been speculated that if the senolytic is an antineoplastic drug, the risk of carcinogenesis is reduced due to the simultaneous removal of oncogenic cells that would otherwise provoke the formation of a tumor.[47]
Small molecules of senolytics
Therapeutics for killing senescent cells could take the form of senolytic small molecules or immune-based clearance (antibodies or cytotoxic T cells).[48] Senescent cells rely on prosurvival stress response adaptations to avoid apoptosis. This suggests that an attractive senescent cell killing approach would be to use small-molecule inhibitors to block cell death-resistance pathways, thereby using the endogenous stress to drive these cells into apoptosis. Existing inhibitors of prosurvival pathways used in cancer therapy may have utility for senescent cell killing, and could be even more effective for this use given that senescent cells, unlike cancer, do not proliferate.
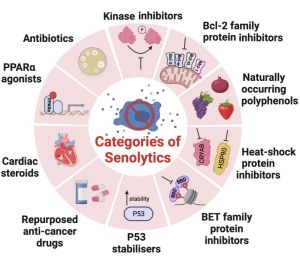
Dasatinib + Quercetin
Dasatinib and Quercertin are a specific combination of medicines (D+Q) used for senescent cell clearance, which began from research in the Mayo Clinic. D and Q have side effects, including hematologic dysfunction, fluid retention, skin rash, and QT prolongation.[50]
Removal of SCs can improve healthspan and lifespan in animal models of premature aging and normal aging. However, some studies suggest that SCs play a fundamental role in physiology and their removal via senolytics or other methods might have deleterious effects in vivo.[51]
The use of one of the senolytics, dasatinib, caused endothelial dysfunction and pulmonary hypertension, which could be corrected using ROCK inhibitors.[52][53]
Treatment with dasatinib has been linked to some uncommon adverse events, such as pleural effusion (PE) and pulmonary arterial hypertension (PAH) Pulmonary arterial hypertension is a life-threatening condition associated with long-term dasatinib therapy, especially in patients with pleural effusion. In the absence of timely treatment, PAH may lead to right ventricular failure. The majority of patients who experienced PAH were female with history or present PE receiving long-term treatment with dasatinib. Animal studies confirmed that dasatinib increased the biological activities of endothelial dysfunction markers (e.g., soluble vascular cell adhesion molecule 1 [VCAM-1], soluble intercellular adhesion molecule 1 [ICAM-1], and soluble E-selectin), leading to minimization of hypoxic vasoconstriction and impairment of endoplasmic reticulum function.[54][55][56]
Studies in mice that also demonstrate impaired tissue repair following clearance of senescent cells raise questions about the potential risks of senolytic therapies. Closer examination of the available studies reveals the hopeful possibility of a ‘therapeutic window’ in which these risks can be minimized.[57]
Use of dasatinib and quercetin has not always been efficacious in every mouse model of metabolic disease, its efficacy seems to be controversial. Although this senolytic cocktail was shown to decrease the burden of senescent cells and reduce hepatic steatosis in one study,[58] it failed to promote clearance of senescent cells and prevent progression of non-alcoholic fatty liver disease in lean mice and in mice with obesity induced by a high-fat diet.
In the pilot study NCT02874989 of the senolytic combination of dasatinib and quercetin (D + Q) for only three weeks in patients with an age-related, chronic idiopathic pulmonary fibrosis (IPF) results suggest that (D + Q) is safe and does not lead to an increase of severe adverse events (AE). However, authors did report on an increase in non-serious AEs, including feeling unwell, cough, nausea, fatigue, weakness, and headache. While these side effects do not pose life-threatening consequences, these complaints could ultimately limit compliance with (D + Q) therapy. For instance, cough is already a problem for many IPF patients and gastrointestinal side effects remain a major factor limiting the tolerability of existing IPF anti-fibrotic treatments.[59]
Long-term study of the intermittent dasatinib plus quercetin (5 mg/kg + 50 mg/kg) exposure (two consecutive days monthly for 6 months) on aging outcomes and inflammation in nonhuman primates resulted in significant positive body composition changes with improvement in immune cell profiles and reduced glycosylated hemoglobin A1c.[60]
A computer-assisted expression analysis study suggested that piperlongumine (a known natural senolytic found in long pepper Piper longum[61]) combination with quercetin (“P+Q”) may be a natural-compound alternative to the combination of dasatinib and quercetin (“D+Q”).[62]
Fisetin
Fisetin is a naturally-occurring flavonoid polyphenol plant dye that is rich in certain fruits and vegetables, such as strawberries, grapes, apples, persimmons, cucumbers, and onions.[63][64] [65]
Fisetin has manifested several health benefits in preclinical models of neurodegenerative diseases such as Alzheimer's disease, Vascular dementia, and Schizophrenia. Parkinson's disease, Amyotrophic Lateral Sclerosis, Huntington's disease, Stroke, Traumatic Brain Injury (TBI), and age-associated changes.[66][67]
Fisetin also demonstrates an anti-diabetic effect through its α-glucosidase inhibitor activity and anti-oxidant activity.[68][69] Fiestin could inhibit the development of diabetic cardiomyopathy by ameliorating hyperglycemia/hyperlipidemia-mediated oxidative stress in STZ rat cardiomyocytes, preventing inflammation and apoptosis, and enhancing the antioxidant capacity.[70] Fisetin inhibits hyperglycemia-induced proinflammatory cytokine production by epigenetic mechanisms.[71] Fisetin has been shown to attenuate obesity and regulate glucose metabolism in a small single-blind, controlled study in Iraq that investigate the effects of 8 weeks of fisetin (100 mg/day) with obese diabetic patients (21 males and 30 females), and could aid as a complementary anti-obesity agent in the treatment of obese diabetic patients.[72]
In aged tissues, fisetin can induce apoptosis specifically in senescent cells and reduce the level of cellular oxidative damage. [73]
Dietary supplementation with fisetin significantly increase both the mean and maximum lifespan in old mice, reducing markers of tissue aging and age-related pathologies even when treatment was initiated in older animals.[65]
In Caenorhabditis elegans fisetin increased the resistance to oxidative stress, but failed to reduce the accumulation of such an aging marker as lipofuscin.[74] However, both the mean and maximum lifespans were significantly extended by fisetin in Caenorhabditis elegans.[75] Lifespan extension by fisetin was accompanied by reduced fertility as a trade-off. Age-related decline in motility was also delayed by supplementation with fisetin.[75] Genetic analysis revealed that lifespan extension by fisetin was mediated by DAF-16-induced stress response and autophagy.[75]
Fisetin showed more enhanced senotherapeutic activity than quercetin in animal and human tissues,[73] and is currently undergoing several clinical trials for multiple age-related diseases, including osteoarthritis (NCT04815902, NCT04210986, NCT04770064), coronavirus infection (NCT04771611, NCT04476953, NCT04537299), frail elderly syndrome (NCT03675724, NCT04733534, NCT03430037), chronic kidney diseases (NCT03325322), and femoroacetabular impingement (NCT05025956). Therefore, the clinical merits of fisetin in terms of feasibility, safety, tolerability, and efficacy could soon be established and employed in geriatric medicine.[76]
Flavonoid 4,4′-dimethoxychalcone
The flavonoid 4,4′-dimethoxychalcone (DMC) is particularly abundant in the plant Angelica keiskei koidzumi, which has been used in Asian traditional medicine, and was documented for its ability to promote autophagy-dependent longevity and health.[77] By inhibiting the enzymatic activity of a metalloenzyme ferrochelatase, DMC induces iron accumulation and further ferroptosis.[78] Since ferrochelatase was highly expressed in senescent cells compared to non-senescent cells DMC inhibited ferrochelatase and induced ferritinophagy, which led to an increase of labile iron pool, triggering ferroptosis of senescent cells.[79]
Curcumin
Although many consider curcumin and its derivatives to be senolytic,[80], there is clear evidence that curcumin does not have selectivity for senescent cells and kills both old and normal cells equally effectively.[81] However, due to principle of synergistic synthetic lethality,[10] its analog EF24 can have a senolytic effect in combination with other senolytics.[82][83]
Zoledronate
Zoledronic acid (ZA) is an effective nitrogen-containing bisphosphonate (NBP), which not only directly induces the apoptosis of tumor cells but also reduces the in vivo amount of tumor-associated macrophages and facilitates the transformation of tumor-associated macrophages into M1 macrophages.[84][85] Large clinical trials found that zoledronate treatment has been associated with ~30% reductions in mortality.[86][87] In vitro, zoledronate exhibited potent senolytic effects with a high selectivity index on both human and mouse senescent cells; (2) in vivo, in aged mice, treatment with zoledronate was associated with a significant reduction in a panel of circulating SASP factors concomitant with an improvement in grip strength.[88]
Anthocyanin
Anthocyanins are natural water-soluble pigments of fruits, and flowers that, due to their antioxidant, anti-inflammatory, antitumoral, and antimicrobial properties are responsible for a plethora of health beneficial functions as dietary antioxidants, that can fight free radicals which raise the risk of chronic diseases onset such as: neuronal disorders, inflammatory conditions, diabetes, obesity, cardiovascular diseases and cancer.[89] The main mechanism by which anthocyanins are believed to have the ability to prevent the development of aging diseases is related to their antioxidant capacity by which they diminish prooxidative damage.[90][91]
Anthocyanin has been shown to inhibit the PI3K/Akt/mTOR signaling pathway of senescent cells, leading to an increase in the ratios of pro-apoptotic to anti-apoptotic proteins Bax/Bcl-2 and Bak/Mcl-1 in anthocyanin-treated cells, suggesting that anthocyanin induces apoptosis in aging cells. These results suggested that anthocyanin might promote the clearance of senescent cells by increasing apoptosis and the proportion of healthy cells. Anthocyanin also enhanced autophagic and mitophagic flux in the senescent cells.[92]
Supramolecular senolytics
Supramolecular senolytics is organic molecules that selectively target receptors overexpressed in the membranes of aging cells. By leveraging the higher levels of reactive oxygen species (ROS) found in aging cells, these molecules promote the formation of disulfide bonds and create oligomers that bind together. Self-assembly of these oligomers occurred only inside the mitochondria of senescent cells due to selective localization of the peptides by cellular uptake into integrin αvβ3-overexpressed senescent cells and elevated levels of reactive oxygen species, which can be used as a chemical fuel for disulfide formation. This oligomerization results in an artificial protein-like nanoassembly with a stable α-helix secondary structure, which can disrupt the mitochondrial membrane via multivalent interactions because the mitochondrial membrane of senescent cells has weaker integrity than that of normal cells. These three specificities (integrin αvβ3, high ROS, and weak mitochondrial membrane integrity) of senescent cells work in combination; therefore, this intramitochondrial oligomerization system can selectively induce apoptosis of senescent cells without side effects on normal cells.[93]
Cycloastragenol
Cycloastragenol, a secondary metabolite isolated from Astragalus membrananceus has a wide spectrum of pharmacological functions, including telomere elongation, telomerase activation, anti-inflammatory effects, antioxidative properties[94] and potent senolytic, which selectively induces cell death in senescent cells via induction of apoptosis by inhibiting the Bcl-2 antiapoptotic family proteins and PI3K/AKT/mTOR pathway. [95] Cycloastragenol also suppresses SASP expression, meaning it can act as a senomorphic to reduce the impact of senescent cells on the age-related phenotype.[95]
Fibrates
Fenofibrate (FN), a PPARα agonist used for dyslipidaemias in humans, reduced the number of senescent cells via apoptosis, increased autophagic flux, and protected against cartilage degradation. FN reduced both senescence and inflammation and increased autophagy in both ageing human and osteoarthritis chondrocytes.[96]
Salvestrols
Salvestrol (lat. salvus - healthy, unharmed) is a very special group of secondary plant substances that are part of the plant’s natural defense system. They are especially formed when the plant is attacked by pathogens. Under the influence of the cytochrome P450 enzyme CYP1B1, which was reported to be involved in performance of two important factors of aging: mitochondrial function and reactive oxygen species (ROS) production,[97] and which is expressed in large quantities in cancer cells[98][99] and due to cellular senescence,[100] salvestrols can be converted into metabolites that cause the death of target cells.[101][102][103]
Plants with a generally higher salvestrol content from organic farming include artichokes, asparagus, watercress, rocket, spinach, pumpkin, olives, currants, apples, rose hip, strawberries, sage, mint, dandelion, plantain, milk thistle, agrimony, lemon verbena, rooibos tea.[104] and especially tangerines.[105]
p53-regulated apoptosis inducers
FOXO4-DRI
The Forkhead box protein O4 D-retro inverso (FOXO4-DRI), a synthetic peptide of D-amino acids in a reversed sequence, leads to senescent cell apoptosis by interrupting the interaction between FOXO4 and p53, leading to release of p53 available to trigger p53 mediated apoptosis. [106] Experiments show that FOXO4-DRI can reduce senescence and features of frailty in a fast aged mice model (XpdTTD/TTD) and also can restore loss of renal function in both naturally and fast aged mice.[106]
In naturally aged mice, FOXO4-DRI improved the testicular microenvironment and alleviated age-related testosterone secretion insufficiency. These findings reveal the therapeutic potential of FOXO4-DRI for the treatment of male late-onset hypogonadism.[107] FOXO4-DRI have also been shown to selectively kill senescent chondrocytes.[108]
UBX0101
UBX-0101 is an experimental senolytic that can selectively remove senescent chondrocytes by inhibiting MDM2/p53 interactions. Despite initial promising results that were seen preclinically,[109] and in the phase 1 trial,[110] no significant difference was observed between the placebo or UBX-0101-treated group of patients with knee osteoarthritis in a phase 2 trial.[111] -
CUDC-907
CUDC-907, a drug already in clinical trials for its antineoplastic effects, that is able to selectively induce apoptosis in cells driven to senesce by p53 expression, but not when senescence happened in the absence of p53.[112] Senolytic functions of CUDC-907 depend on the inhibitory effects of both histone deacetylase (HDAC) and phosphoinositide 3-kinase (PI3K), which leads to an increase in p53 and a reduction in BH3 (the Bcl-2 homology (BH) domain necessary for dimerization with other proteins of Bcl-2 family) pro-survival proteins.[112]
UBX1325
UBX1325, a small molecule inhibitor of specific subtypes within the B-cell lymphoma 2 (Bcl-2) family of apoptosis regulatory proteins and assessed its efficacy in senescence-associated models of retinopathy. Inhibition of retinal Bcl-xL by UBX1325 promotes apoptosis in the senescence-associated oxygen induced retinopathy model.[113]
A single intravitreal injection of UBX1325 up to 10 μg was safe and well tolerated in patients with advanced Diabetic macular edema or wet age-related macular degeneration, through 24 weeks.[114]
Macrolide antibiotics
Two macrolide antibiotics, azithromycin and roxithromycin, belonging to the erythromycin family, have shown themselves to be senolytics. Unlike erythromycin itself, these acid-resistant analogues, in in vitro tests with aged fibroblasts, removed approximately 97% of aged cells and thus reduced the number of aged cells by a factor of 25.[115][116] They seem to be able to act in a similar way in the body, as roxithromycin (and to a lesser extent azithromycin) is known to have powerful anti-inflammatory abilities, reducing the level of cytokines in the body,[117][118][119] and promoting of tissue repair.[120] However, systemic administration of azithromycin or roxithromycin has been associated with many adverse effects including cardiotoxicity.[121] In addition, there is a risk of the emergence of macrolide resistance with the prolonged administration for other chronic lung conditions.[122] In the light of this, novel therapeutic strategies, including the encapsulation of azithromycin or roxithromycin using nanocapsules that preferentially introduce the senolytic toxin specifically to target senescent cells of lungs must be employed, such as nanoformulations suitable for inhalation.[123][124] In particular, the inhalation of Azithromycin Nanoformulation at a low dose of 11 mg/kg, markedly alleviated the pro-inflammatory markers (IL-6, IL-1β, TNF-α, and NF-kB), the ones that were high in the pulmonary tissues of the model of acute lung injury.[123]
It would be interesting to check also the aptness to the destruction of senescent cells by a non-antibiotic macrolide, EM900, which, like azithromycin, has an anti-inflammatory ability.[125]
Navitoclax (ABT-263), is an anticancer agent, that induces apoptosis in senescent cells by inhibiting the activities of Bcl-2, Bcl-xL, and BcL-w[126][127]
ABT-263 can be used to exclusively eliminate senescent cells, since transcriptome analysis showed that the inhibition of apoptosis through the upregulation of the Bcl family proteins was specific to senescent cells and not found in young cells.[26] ABT-263 has been shown to attenuate the development of pulmonary fibrosis.[128][126]
ABT-263 treatment of aged skin from men clearly resulted in rejuvenation through the clearance of senescent cells and inhibition of the secretion and inflammatory state of the senescence-associated secretory phenotype (SASP), compared with that in the original skin or control groups.[129]
ABT263 inhibited the formation of osteoclasts and had a significant therapeutic effect on mouse cranial osteolysis.[130]
PROTAC technology
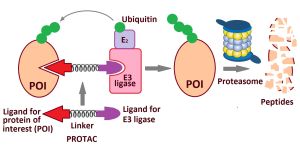
Proteolysis-targeting chimeras (PROTACs) are an innovative technology to induce degradation of a protein of interest (POI).[132] PROTACs are composed of three elements: a ligand that binds to a target POI, an E3 ligase recruiting ligand, and a flexible linker between the two ligands. Thus, a PROTAC can form a stable ternary complex with a POI and E3 ligase, resulting in subsequent ubiquitination and proteasomal degradation of the POI. The PROTAC is then recycled to attack another copy of the POI. This catalytic mode of action eliminates the need to maintain high drug levels, both characteristics that distinguish PROTACs from classical occupancy-driven pharmacology of small-molecule inhibitors.[133] PROTACs have several advantages, such as increased potency, higher selectivity, prolonged activity, and reduced toxicity, which make them an attractive strategy for developing senotherapeutics.[134]
Aptamers are short oligonucleotides (DNA/RNA) or peptide molecules that can selectively bind to their specific targets with high specificity and affinity.[135] Aptamers, as therapeutic agents, can effectively recognize various proteins on the cell membrane or in the blood circulation to modulate their interaction with receptors and affect the corresponding biological pathways for the treatment of aging and various diseases. Owing to remarkable specificity and binding affinity, aptamers can be utilized as target molecules for the construction of PROTAC that is able to degrade target disease or aging-causing proteins.[136][137][138][139]
In particular, an aptamer-senolytic molecular prodrug was developed for reliable regulation of vascular senescence through hierarchical recognition of three types of senescence-related hallmarks commonly shared among senescence, namely, aptamer-mediated recognition of a membrane marker for active cell targeting, a self-immolative linker responsive to lysosomal enzymes for switchable drug release, and a compound against antiapoptotic signaling for clearance. According to preliminary data, it can actively target and trigger cell-specific apoptosis in senescent endothelial cells caused by various stimuli, while keeping silent in non-senescent cells, contributing to effective inhibition effects on the senescence burden-induced progress of atherosclerosis. Such senolytic can target and trigger severe cell apoptosis in broad-spectrum senescent endothelial cells, and importantly, distinguish them from the quiescent state.[140]
BET degraders as senolytic drugs
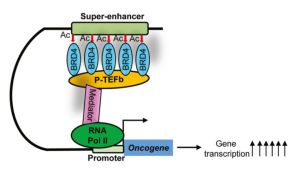
Super-enhancers are large clusters of enhancers that are in close genomic proximity, are densely bound by the BET bromodomain protein BRD4 and master transcription factors, and are characterized by massive H3K27ac and H3K4me signals in ChIP sequencing (Chromatin immunoprecipitation followed by sequencing).[142] Super-enhancers and their reader BRD4 are critical tumorigenic drivers.[141]
Expression of bet-1, the C. elegans ortholog of human BRD2 and BRD4, directly impacts actin organization and function, which has direct significance in longevity. Specifically, loss of function of bet-1 results in premature breakdown of actin structure during aging, while its overexpression protects actin filaments at late age and promotes both healthspan and life span. Importantly, that these effects are conserved in human cells, as inhibition of BRD4 in non-dividing, human senescent cells result in decreased actin filaments, decreased adhesion, and decreased cell survival.[143] Senescent cells require a stabilized actin network to maintain adherence, which is critical for cell survival.[144]
Hetero bifunctional molecule, ARV-825, that cause cleavage and degradation of BET proteins, was designed by connecting a small molecule BRD4 binding moiety (OTX015) to an E3 ligase cereblon binding moiety (pomalidomide) using PROTAC technology.[145]
Unlike previously reported senolytic drugs, ARV825 exhibits robust senolysis activity even at nanomolar concentrations (5–10 nM). The optimum concentration (10 nM) of ARV825 for senolysis does not provoke cell death in quiescent cells. However, a treatment with a high concentration (more than 50 nM) of ARV825 reduce the proliferation of cells. So, it is crucial to determine the optimal concentration of ARV825 in vivo.[146] In an experimental mouse model of lung fibrosis, ARV825 attenuated lung fibrosis and improved lung function. Immunohistochemical staining revealed a significant decrease in the number of senescent alveolar type 2 cells in lung tissue due to ARV825 treatment.[147]
BETd-246, a BRD degrader belonging to the second generation, exhibits favorable selectivity and anti-neoplastic properties. BETd-246 exhibits significant therapeutic efficacy against lung cancer and hematological cancer.[148] BRD4 is also a repressor in cardiac reprogramming, acting primarily through cytokine oncostatin-M, and transient, but not permanent, degradation of BRD4 by a BET degrader, senolytic BETd-246 treatment can enhance cardiac-reprogramming-based regeneration in vivo.[149]
PZ15227
PZ15227 was generated by tethering of the senolytic drug navitoclax (ABT-263) to a cereblon (CRBN) E3 ligand that is expressed minimally in normal platelets.[150] PZ15227 binds to BCL-XL, causing it to be degraded by the cereblon (CRBN) E3 ligase. Compared with ABT263, PZ15227 was shown to be less toxic to platelets, but was a more potent senolytic in vitro and in vivo.[151]
DT2216
DT2216 an effective BCL-XL degrader based on VHL E3 ligase. DT2216 exerted almost no effect on the viability of platelets up to a concentration of 3 μM which showed better effect than PZ15227. DT2216 was found to have enhanced efficacy against a variety of BCL-XL-dependent leukemia cell lines and exhibited much less toxic to platelets than ABT263.[152] Therefore, DT2216 was approved by FDA to enter phase I clinical trials for the treatment of advanced liquid and solid tumors.
Inhibitors of CRYAB
Crystallin Alpha B (CRYAB or HspB5) is a stress-induced small (20-kd) heat-shock protein highly expressed in the lens and to a lesser extent in several other tissues, among which heart, skeletal muscle and brain.[153] CRYAB acts as a molecular chaperone involved in protein folding and is associated with apoptosis in cardiovascular disease.[154] As a member of the HSPB family and an important molecular chaperone, HSPB5 is involved in cytoskeleton stability, growth and differentiation, proliferation and cell migration and is closely related to the occurrence and development of a variety of diseases.[155][156][157] In particular, its overexpression can promote tumorigenesis and metastasis.[158][159]
It was found that in living organisms a powerful senolytic is produced that can cause lysis of aged cells by acting on CRYAB, and this senolytic turned out to be 25-hydroxycholesterol (25HC), which is an endogenous metabolite of cholesterol biosynthesis.[160] 25HC targets CRYAB in many cell types, including the lung, and is localized in alveolar macrophages and pneumocytes of COPD patients. 25HC is the only oxysterol induced by bacterial endotoxin lipopolysaccharides (LPS) in the lung and its induction requires enzymatic activity of cholesterol 25-hydroxylase (CH25H) in macrophages.[161] So, inhibitors of CRYAB can lead to potent senolysis, and 25-hydroxycholesterol (25HC) represents a potential class of senolytics, which may be useful in combating diseases or physiologies in which cellular senescence is a key driver. However, it should be borne in mind that the elevated 25HC may contribute to fibroblasts-mediated lung tissue remodeling by promoting myofibroblasts differentiation and the excessive release of matrix metalloproteinases through the NF-kB-TGF-β-dependent pathway.[162]
Ginkgetin, oleandrin and periplocin
Predicting of senolytic compounds by computational screening using machine learning made it possible to find new potential senolytics, including ginkgetin, oleandrin and periplocin.[163] Of the three, oleandrin was found to be the most effective.
Activatable senolytics
Selective senolytic platform SenTech™ of Rubedo Life Sciences
Many known senolytic agents were initially developed as cytotoxic anti-cancer agents and subsequently repurposed for ‘selective’ removal of senescent cell populations. Because proliferating cells are frequently more sensitive to the cytotoxic or cytostatic effect of anti-tumor agents, dose-limiting toxicity, especially in rapidly replicating hematopoietic, skin or gut cells, is a frequently observed side-effect, which strongly limits the clinical utility of these anti-senescence therapies. To minimize the side effects of senolytics, it is necessary to identify senolytics that can be targeted to senescent cells safely, selectively and systemically. The most frequently used assays (e.g. immune-histochemistry or flow cytometry-based) for identifying senescent cells measure the levels of senescence-associated β-galactosidase (SA-β-gal), which is present at a low level in all cells but is substantially increased in senescent cells.[38] Biopharmaceutical company Rubedo Life Sciences has presented its small molecule therapy allowing systemic removal of senescent cells in geriatric mice without noticeable side effects. Based on galactose-derivative prodrug 5-fluorouridine-5′-O-β-Dgalactopyranoside (5FURGal) it can, upon selective activation in senescent cells by the hydrolase activity of SA-βGal, release clinically approved anti-cancer medication 5-Fluorouracil.[164] Geriatric (30 month old) mice that received the prodrug treatment for four weeks altogether improved significantly: 1) frailty profile; 2) skeletal muscle function; 3) muscle stem cell function; 4) cognitive function; and 5) survival.[164] Similar results have been obtained with other such drugs.[165][166]
Photoablation of senescent cells
Light as an external medical stimulus is an easy and convenient tool useful for noninvasive therapy. Therefore, a photosensitive senolytic prodrug KSL0608-Se was created for photoablation of senescent cells, which uses "a combination of the enzyme substrate of senescence-associated β-galactosidase (SA-β-gal) with fluorescence tag for the precise tracking of senescent cells, construction of a bioorthogonal receptor triggered by SA-β-gal to target and anchor senescent cells with single-cell resolution and incorporation of a selenium atom to generate singlet oxygen and achieve precise senolysis through controllable photodynamic therapy". So, KSL0608-Se, is a photosensitive senolytic prodrug, which is selectively activated by SA-β-gal.[167] In naturally-aged mice, KSL0608-Se-mediated photodynamic therapy prevented upregulation of age-related senescent markers and senescence-associated secretory phenotype factors. This treatment also countered age-induced losses in liver and renal function and inhibited the age-associated physical dysfunction in mice.[167]
Future target senolytics
The atypical chemokine receptor 3 (ACKR3), is a cell surface protein, the membrane surface receptor of CXCL12 (CXC motif chemokine 12) that is specifically present in senescent cells but not on proliferating cells.[168] CXCL12 is known to be central to the development of many organs and later on involved in pathophysiological processes underlying cancer, inflammation, and cardiovascular disorders.[169] The selective expression of ACKR3 on the surface of senescent cells allows the preferential elimination of senescent cells and might contribute to the future development of novel senolysis approaches..[168]
Developments
The SENSOlytic platform is Oisín's patented technology that selectively removes senescent cells based on p16 gene expression in senescent cells rather than surface markers or other characteristics that may be shared with normal, intact cells. Oisín has developed a therapeutic delivery device that it calls a proteo-lipid vehicle that carries inside of it DNA and can be injected into patients. The vehicle fuses with a patient’s cells and releases its DNA payload into them. When it connects with a target cell — perhaps a senescent or cancerous cell — the DNA triggers its death. The startup has been testing the technology in mice. Treated mice lived 20% longer even when treatment was started in old age, and after a single treatment, senescent cell removal rates reached as high as 70%.[171] So, the cell is killed by an exogenous gene that causes apoptosis (presumably caspase 9), which is activated only in cells where the p16 gene is active. Delivery of this gene into the cell is carried out by a lipid nanoparticle (artificial liposome) containing DNA with a gene that causes apoptosis.
Garcia H. et al., describe a clinically viable gene therapy consisting of a suicide gene under a senescent cell promoter delivered in vivo with Proteo-Lipid Vehicles (PLVs). These PLVs employ fusion-associated small transmembrane (FAST) proteins that can efficiently transduce a wide range of cells in vivo. Selective ablation of target cells is then achieved through the expression of a potent pro-apoptotic transgene driven by a specific senescence-associated promoter such as p16Ink4A or p53. Aged mice treated with FAST-PLV senolytic showed significantly reduced senescent cell burden. Mice treated with senolytic PLVs had an increased median post-treatment survival of 160%, lower clinical frailty, and improved physical and heart function. Spontaneous tumor burden in these mice was reduced.[172]
Senolytic CAR T cells and natural killer (NK) cells
Senescence in the immune compartment, as occurs with normal ageing, affects innate and adaptive immunity, in particular natural killer cell function, which cleanse the body of old inoperable cells, and potently drives senescence and age-related changes in solid organs.[32][173] Diminished Natural killer (NK) cells activity in elderly individuals is associated with disorders such as atherosclerosis, the development of hypertension[174][175] and an elevated risk of mortality.[176][177][178]
Development of the CAR-T cells directed against a senescence-specific surface antigens has opened a new and very specific alternative to directly target pathological cells.[179][180] For example, in mice with cardiac fibrosis, adoptive transfer of T cells expressing a CAR against the fibroblast activation protein effectively reduced fibrosis and restored cardiac function after injury. The use of CAR immunotherapy offers a potential alternative to current therapies for fibrosis reduction and restoration of cardiac function in patients with myocardial fibrosis.[181][182] Because natural killer group 2 member D ligands (NKG2DLs) are up-regulated in senescent cells, NKG2D-CAR T cells could serve as potent and selective senolytic agents for aging and age-associated diseases driven by senescence. Сhimeric antigen receptor (CAR) T cells targeting human NKG2DLs selectively and effectively diminish human cells undergoing senescence induced by oncogenic stress, replicative stress, DNA damage, or p16INK4a overexpression in vitro. Targeting senescent cells with mouse NKG2D-CAR T cells alleviated multiple aging-associated pathologies and improved physical performance in both irradiated and aged mice. Autologous T cells armed with the human NKG2D CAR effectively delete naturally occurring senescent cells in aged nonhuman primates without any observed adverse effects.[183]
Barriers to using this technology in the clinic are that clinical production of CAR-T cells is still complex, expensive and time-consuming, and because of adverse effects such as cytokine release syndrome (CRS), caused by the massive release of proinflammatory cytokines by activated T cells and other immune cells. In addition, exogenously produced CAR-T cells are usually short-lived after repeated injections into the recipient.[184] To overcome this, a technology has been created for the production of CAR-T cells directly in vivo. According to this technology, for the treatment of cardiac fibrosis after heart injury, mice were injected with lipid nanoparticles (LNPs) targeting to T cells through the expression of anti-CD5 (a T-cell marker) carrying a modified mRNA encoding a CAR against fibroblast activated protein. The in vivo generated CAR-T cells exerted anti-fibrotic properties and restored cardiac function in mice, holding promising therapeutic potential in a wide range of diseases progressing with fibrosis[185] The LNP-mRNA delivery system has advantages including having no integration in host genome, inexpensiveness, low toxicity and modifiability; on the other hand, it has certain disadvantages such as limited cell persistence caused by transient protein expression and limitations in preparation techniques.[186][187]
Senolytic therapy based on chimeric antigen receptor (CAR) T cells targeting the senescence-associated protein urokinase plasminogen activator receptor (uPAR) can safely eliminate uPAR-positive senescent cells that accumulate during aging.[179] Treatment with anti-uPAR CAR T cells improves exercise capacity in physiological aging, and it ameliorates metabolic dysfunction (for example, improving glucose tolerance) in aged mice and in mice on a high-fat diet. Importantly, a single administration of these senolytic CAR T cells is sufficient to achieve long-term therapeutic and preventive effects.[188]
Alternatively, NK-cell-based therapies show promise in rejuvenating immunosenescence, eliminating senescent cells and alleviating SASPs, that lead to aging-associated diseases.[189] The rapid development of inexpensive and accessible non-viral methods for engineering immune cells makes this approach a promising way to combat diseases of aging.[190]
Senolytic vaccination
Analysis of transcriptome data from senescent vascular endothelial cells revealed that glycoprotein nonmetastatic melanoma protein B (GPNMB) was a molecule with a transmembrane domain that was enriched in senescent cells (seno-antigen). Near-end-of-lifespan (27 months) wild-type mice have 35-fold increased hepatic levels of Gpnmb in comparison to young (4 months) mice. GPNMB expression was also upregulated in vascular endothelial cells and/or leukocytes of patients and mice with atherosclerosis.[191][192] Immunization of mice against GNMPB reduced the burden of senescent cells, improved the healthspan of naturally aged mice, and prolonged the lifespan of Zmpste24 knockout progeroid mice.[192] The vaccine reduces atherosclerotic plaque burden and metabolic dysfunction such as glucose intolerance in mouse models of obesity and atherosclerosis.[192] For translation to humans the activity of the vaccine will need to be tightly controlled, as the target GPNMB has multiple roles in normal physiology, including acting to inhibit and possibly resolve inflammation.[191] A promising alternative approach would be to use passive immunization with a monoclonal antibody directed against GPNMB.[193]
The proteins and pathways involved in senescent cells apoptotic resistance
Elimination of senescent cells has the potential to delay aging, treat age-related diseases and extend healthspan.[194] However, once cells becoming senescent, they are more resistant to apoptotic stimuli.[195][196] At least 125 different genes are involved in the aging process,[197][17][198] a set of which, called “SenMayo”, makes it possible to identify old cells.[199][200] Due to the high heterogeneity in gene expression and their diverse origins, senescent cells may use different anti-apoptotic pathways to maintain their survival, making it difficult to use a single senolytic to kill all types of senescent cells.[201][202]
Apoptosis
Aging has been associated with decreased apoptosis in most cell types, which acts as an important contributor to aging, and age-related diseases, since high resistance to apoptosis allows functionally deficient, post-mitotic senescent cells to accumulate during aging.[203] Prolonged persistence of senescent cells is associated with tissue dysfunction and pathology.[204] The key executioners of apoptosis are proteases called caspases; when caspases are activated, apoptosis becomes irreversible.[205] Caspase activation is tightly controlled by regulatory molecules, including such highly conserved regulators as protein families Bcl-2 and the inhibitor of apoptosis (IAP) proteins.[206][207] IAPs are characterized by the presence of baculoviral repeat domain (BIR) repeats and are recruited into signaling complexes which function as ubiquitin E3 ligases, via their RING (really interesting new gene) domains.[208] In addition to cell death, IAPs also act as innate immune sensors and modulate multiple pathways, such as autophagy and cell division.[209]
IAPs are regulated by mitochondria-derived pro-apoptotic factors such as Smac (second mitochondria-derived activator of caspases)[210] and heat shock protein HtrA2 (high-temperature requirement A2) peptidase.[211] Each of them can bind IAPs, thus freeing caspases to activate apoptosis.[212] The BIR domain found in all IAPs interacts with the conserved IAP binding motif (IBM) of caspases. Similar IBMs are found on Smac and HtrA2.[213]
In particular, the ubiquitin ligase BIRC6 (baculoviral IAP repeat–containing protein 6) inhibit apoptosis by binding to apoptotic proteases, keeping them inactive and targeting these proteins for degradation, preventing cell death.[214] BIRC6 adopts a dimeric, horseshoe-shaped architecture with a central cavity that allows for binding to target proteases. The pro-apoptotic protein Smac binds very tightly to the same interior site as the proteases through multiple interactions, essentially irreversibly blocking the ability of BIRC6 to bind substrates.[215][204]
References
- ↑ Kirkland, J. L., & Tchkonia, T. (2020). Senolytic drugs: from discovery to translation. Journal of internal medicine, 288(5), 518-536. PMID: 32686219 PMC link DOI: 10.1111/joim.13141
- ↑ Baker, D. J., Wijshake, T., Tchkonia, T., LeBrasseur, N. K., Childs, B. G., Van De Sluis, B., ... & Van Deursen, J. M. (2011). Clearance of p16Ink4a-positive senescent cells delays ageing-associated disorders. Nature, 479(7372), 232-236. PMID: 22048312 PMC link DOI: 10.1038/nature10600
- ↑ Ageing: The Biology of Senescence. By Alex Comfort. Pp. xvi + 365 London: Routledge and Kegan Paul, 1964. Second Edition.
- ↑ Calabrese, E. J. (2014). Hormesis: a fundamental concept in biology. Microbial cell, 1(5), 145. PMID: 28357236 PMC link DOI: 10.15698/mic2014.05.145
- ↑ Lin, C. L. (2022). Mitophagy and mitohormetics: Promising antiaging strategy. In Anti-Aging Drug Discovery on the Basis of Hallmarks of Aging (pp. 279-289). Academic Press. https://doi.org/10.1016/B978-0-323-90235-9.00001-X
- ↑ Iyanu Oduwole, A. A. (2020). Amygdalin-therapeutic effects and toxicity. Journal of Biotechnology and Biomedicine, 3(2), 39-49.
- ↑ Saberi-Hasanabadi, P., & Shaki, F. (2022). The Pharmacological Activities and Toxicological Effects of Amygdalin: A Review. Pharmaceutical and Biomedical Research, 8(1), 1-12. http://pbr.mazums.ac.ir/article-1-387-en.html
- ↑ Percy, C. (1974). You Live to Be 100 in Hunza. Parade, 11.
- ↑ Simons, A., Dafni, N., Dotan, I., Oron, Y., & Canaani, D. (2001). Establishment of a chemical synthetic lethality screen in cultured human cells. Genome research, 11(2), 266-273. PMID: 11157789 PMC link DOI: 10.1101/gr.154201
- ↑ 10.0 10.1 Tozaki, Y., Aoki, H., Kato, R., Toriuchi, K., Arame, S., Inoue, Y., ... & Aoyama, M. (2023). The Combination of ATM and Chk1 Inhibitors Induces Synthetic Lethality in Colorectal Cancer Cells. Cancers, 15(3), 735. PMID: 36765693 PMC link DOI: 10.3390/cancers15030735
- ↑ 11.0 11.1 Marques-Reis, M., & Moreno, E. (2021). Role of cell competition in ageing. Developmental Biology, 476, 79-87. PMID: 33753080 DOI:link
- ↑ Reed, R., & Miwa, S. (2023). Cellular Senescence and Ageing. In Biochemistry and Cell Biology of Ageing: Part III Biomedical Science (pp. 139-173). Cham: Springer International Publishing. PMID: 36600133 DOI:link
- ↑ Borghesan, M., Hoogaars, W. M. H., Varela-Eirin, M., Talma, N., & Demaria, M. (2020). A senescence-centric view of aging: implications for longevity and disease. Trends in Cell Biology, 30(10), 777-791. PMID: 32800659 DOI:link
- ↑ Van Deursen, J. M. (2014). The role of senescent cells in ageing. Nature, 509(7501), 439-446. PMID: 24848057; PMCID: PMC4214092 link] doi: 10.1038/nature13193
- ↑ 15.0 15.1 Scudellari, M. (2017). To stay young, kill zombies. Nature, 550(7677), 448-450. PMID: 29072283 DOI:link
- ↑ Muñoz-Espín, D., & Serrano, M. (2014). Cellular senescence: from physiology to pathology. Nature reviews Molecular cell biology, 15(7), 482-496. PMID: 24954210 DOI:link
- ↑ 17.0 17.1 Gorgoulis, V., Adams, P. D., Alimonti, A., Bennett, D. C., Bischof, O., Bishop, C., ... & Demaria, M. (2019). Cellular senescence: defining a path forward. Cell, 179(4), 813-827. PMID: 31675495 DOI:link
- ↑ 18.0 18.1 18.2 Reyes, N. S., Krasilnikov, M., Allen, N. C., Lee, J. Y., Hyams, B., Zhou, M., ... & Peng, T. (2022). Sentinel p16 INK4a+ cells in the basement membrane form a reparative niche in the lung. Science, 378(6616), 192-201.
- ↑ 19.0 19.1 19.2 19.3 Zhang, L., Pitcher, L. E., Yousefzadeh, M. J., Niedernhofer, L. J., Robbins, P. D., & Zhu, Y. (2022). Cellular senescence: a key therapeutic target in aging and diseases. Journal of Clinical Investigation, 132(15), e158450. PMID: 35912854 PMC link DOI: 10.1172/JCI158450
- ↑ João Pedro de Magalhães (2024). Cellular senescence in normal physiology.Science, 384, 1300-1301. DOI:10.1126/science.adj7050
- ↑ Idda, M. L., McClusky, W. G., Lodde, V., Munk, R., Abdelmohsen, K., Rossi, M., & Gorospe, M. (2020). Survey of senescent cell markers with age in human tissues. Aging (Albany NY), 12(5), 4052. PMID: 32160592 PMC link DOI: 10.18632/aging.102903
- ↑ Demaria, M., Ohtani, N., Youssef, S. A., Rodier, F., Toussaint, W., Mitchell, J. R., ... & Campisi, J. (2014). An essential role for senescent cells in optimal wound healing through secretion of PDGF-AA. Developmental cell, 31(6), 722-733. PMID: 25499914 PMC link DOI: 10.1016/j.devcel.2014.11.012
- ↑ Ritschka, B., Storer, M., Mas, A., Heinzmann, F., Ortells, M. C., Morton, J. P., ... & Keyes, W. M. (2017). The senescence-associated secretory phenotype induces cellular plasticity and tissue regeneration. Genes & development, 31(2), 172-183. PMID: 28143833 PMC link DOI: 10.1101/gad.290635.116
- ↑ Walters, H., Troyanovskiy, K., & Yun, M. H. (2023). Senescent cells enhance newt limb regeneration by promoting muscle dedifferentiation. Aging Cell, 22(6), e13826 https://doi.org/10.1111/acel.13826
- ↑ Lemaitre, J. M. (2024). Looking for the philosopher's stone: Emerging approaches to target the hallmarks of aging in the skin. Journal of the European Academy of Dermatology and Venereology, 38, 5-14.https://doi.org/10.1111/jdv.19820
- ↑ 26.0 26.1 Zhu, Y. I., Tchkonia, T., Pirtskhalava, T., Gower, A. C., Ding, H., Giorgadze, N., ... & Kirkland, J. L. (2015). The Achilles’ heel of senescent cells: from transcriptome to senolytic drugs. Aging cell, 14(4), 644-658. PMID: 25754370 PMC link DOI: 10.1111/acel.12344
- ↑ Liu, H., Zhao, H., & Sun, Y. (2022). Tumor microenvironment and cellular senescence: Understanding therapeutic resistance and harnessing strategies. In Seminars in Cancer Biology (Vol. 86, pp. 769-781). Academic Press. PMID: 34799201 DOI:link
- ↑ Kim, H. J., Kim, G., Lee, J., Lee, Y., & Kim, J. H. (2022). Secretome of stem cells: roles of extracellular vesicles in diseases, stemness, differentiation, and reprogramming. Tissue Engineering and Regenerative Medicine, 1-15. PMID: 34817808 PMC link DOI: 10.1007/s13770-021-00406-4
- ↑ Urman, M. A., John, N. S., & Lee, C. (2023). Age-dependent structural and morphological changes of the stem cell niche disrupt spatiotemporal regulation of stem cells and drive tissue disintegration. bioRxiv, 2023-01. Doi: 10.1101/2023.01.15.524122
- ↑ Lucas, V., Cavadas, C., & Aveleira, C. A. (2023). Cellular senescence: from mechanisms to current biomarkers and senotherapies. Pharmacological Reviews. PMID: 36732079 DOI:link
- ↑ DiLoreto, R., & Murphy, C. T. (2015). The cell biology of aging. Molecular biology of the cell, 26(25), 4524-4531. PMID: 26668170 PMC link DOI: 10.1091/mbc.E14-06-1084
- ↑ 32.0 32.1 Yousefzadeh, M. J., Flores, R. R., Zhu, Y. I., Schmiechen, Z. C., Brooks, R. W., Trussoni, C. E., ... & Niedernhofer, L. J. (2021). An aged immune system drives senescence and ageing of solid organs. Nature, 594(7861), 100-105. PMID: 33981041 PMC link DOI: 10.1038/s41586-021-03547-7
- ↑ Muñoz-Espín, D., & Serrano, M. (2014). Cellular senescence: from physiology to pathology. Nature reviews Molecular cell biology, 15(7), 482-496. PMID: 24954210 DOI:link
- ↑ Maruyama, T., & Fujita, Y. (2022). Cell competition in vertebrates—a key machinery for tissue homeostasis. Current Opinion in Genetics & Development, 72, 15-21. PMID: 34634592 DOI:link
- ↑ Merino, M. M. (2023). Azot expression in the Drosophila gut modulates organismal lifespan. Communicative & Integrative Biology, 16(1), 2156735. PMID: 36606245 PMC link DOI: 10.1080/19420889.2022.2156735
- ↑ Yusupova, M., & Fuchs, Y. (2023). To not love thy neighbor: mechanisms of cell competition in stem cells and beyond. Cell Death & Differentiation, 30(4), 979-991. PMID: 36813919 PMCID: PMC10070350 (available on 2024-04-01) DOI:10.1038/s41418-023-01114-3
- ↑ Yusupova, M., Ankawa, R., Yosefzon, Y., Meiri, D., Bachelet, I., & Fuchs, Y. (2023). Apoptotic dysregulation mediates stem cell competition and tissue regeneration. Nature Communications, 14(1), 7547. PMC10662150 PMID:37985759 DOI:10.1038/s41467-023-41684-x
- ↑ 38.0 38.1 Dimri, G. P., Lee, X., Basile, G., Acosta, M., Scott, G., Roskelley, C., ... & Pereira-Smith, O. (1995). A biomarker that identifies senescent human cells in culture and in aging skin in vivo. Proceedings of the National Academy of Sciences, 92(20), 9363-9367. PMID: 7568133 PMC link DOI: 10.1073/pnas.92.20.9363
- ↑ Englund, D. A., Jolliffe, A., Aversa, Z., Zhang, X., Sturmlechner, I., Sakamoto, A. E., ... & LeBrasseur, N. K. (2023). p21 induces a senescence program and skeletal muscle dysfunction. Molecular metabolism, 67, 101652. PMID: 36509362 PMC link DOI: 10.1016/j.molmet.2022.101652
- ↑ Evangelou, K., Lougiakis, N., Rizou, S. V., Kotsinas, A., Kletsas, D., Muñoz‐Espín, D., ... & Gorgoulis, V. G. (2017). Robust, universal biomarker assay to detect senescent cells in biological specimens. Aging cell, 16(1), 192-197. PMID: 28165661 PMC link DOI: 10.1111/acel.12545
- ↑ Berardi, D., Farrell, G., Al Sultan, A., McCulloch, A., Rattray, Z., & Rattray, N. J. (2022). Integration of mass-spectrometry-based metabolomics and proteomics to characterise different senescence induced molecular sub-phenotypes. bioRxiv, 2022-11. https://doi.org/10.1101/2022.11.30.518588
- ↑ Baker, D. J., Wijshake, T., Tchkonia, T., LeBrasseur, N. K., Childs, B. G., Van De Sluis, B., ... & Van Deursen, J. M. (2011). Clearance of p16Ink4a-positive senescent cells delays ageing-associated disorders. Nature, 479(7372), 232-236. PMID: 22048312 PMC link DOI: 10.1038/nature10600
- ↑ Baker, D. J., Childs, B. G., Durik, M., Wijers, M. E., Sieben, C. J., Zhong, J., ... & Van Deursen, J. M. (2016). Naturally occurring p16Ink4a-positive cells shorten healthy lifespan. Nature, 530(7589), 184-189. PMID: 26840489 PMC link DOI: 10.1038/nature16932
- ↑ Guzman, S. D., Judge, J., Shigdar, S. M., Paul, T. A., Davis, C. S., Macpherson, P. C., ... & Brooks, S. V. (2022). Removal of p16INK4 expressing cells in late life has moderate beneficial effects on skeletal muscle function in male mice. Frontiers in Aging, 2, 85. PMID: 35821997 PMC link DOI: 10.3389/fragi.2021.821904
- ↑ Merino, M. M., Rhiner, C., Lopez-Gay, J. M., Buechel, D., Hauert, B., & Moreno, E. (2015). Elimination of unfit cells maintains tissue health and prolongs lifespan. Cell, 160(3), 461-476. PMID: 25601460 PMC link DOI: 10.1016/j.cell.2014.12.017
- ↑ Dungan, C. M., Murach, K. A., Zdunek, C. J., Tang, Z. J., VonLehmden, G. L., Brightwell, C. R., ... & Peterson, C. A. (2022). Deletion of SA β‐Gal+ cells using senolytics improves muscle regeneration in old mice. Aging Cell, 21(1), e13528. PMID: 34904366 PMC link DOI: 10.1111/acel.13528
- ↑ López-Otín, C., Pietrocola, F., Roiz-Valle, D., Galluzzi, L., & Kroemer, G. (2023). Meta-hallmarks of aging and cancer. Cell Metabolism, 35(1), 12-35. PMID: 36599298 DOI:link
- ↑ Massoud, G. P., Eid, A. E., Booz, G. W., Rached, L., Yabluchanskiy, A., & Zouein, F. A. (2023). Senolytics in diseases: killing to survive. In Anti-Aging Pharmacology (pp. 245-267). Academic Press. https://doi.org/10.1016/B978-0-12-823679-6.00009-6
- ↑ Power, H., Valtchev, P., Dehghani, F., & Schindeler, A. (2023). Strategies for senolytic drug discovery. Aging Cell, e13948. PMID: 37548098 DOI: 10.1111/acel.13948
- ↑ Breccia, M., Molica, M., & Alimena, G. (2014). How tyrosine kinase inhibitors impair metabolism and endocrine system function: a systematic updated review. Leukemia research, 38(12), 1392-1398. PMID: 25449685 DOI:link
- ↑ Born, E. et al. (2022) “Eliminating senescent cells can promote pulmonary hypertension development and progression,” Circulation[Preprint]. Available at: https://doi.org/10.1161/circulationaha.122.058794.
- ↑ Fazakas, C., Nagaraj, C., Zabini, D., et al., & Bálint, Z. (2018). Rho-kinase inhibition ameliorates dasatinib-induced endothelial dysfunction and pulmonary hypertension. Frontiers in physiology, 9. 9: 537 doi: 10.3389/fphys.2018.00537 PMC link
- ↑ Nekoukar, Z., Moghimi, M., & Salehifar, E. (2021). A narrative review on adverse effects of dasatinib with a focus on pharmacotherapy of dasatinib-induced pulmonary toxicities. Blood research, 56(4), 229-242. PMID: 34776414 PMC link DOI: 10.5045/br.2021.2021117
- ↑ Guignabert, C., Phan, C., Seferian, A., Huertas, A., Tu, L. Y., Thuillet, R., ... & Humbert, M. (2016). Dasatinib induces lung vascular toxicity and predisposes to pulmonary hypertension. The Journal of clinical investigation, 126(9), 3207-3218. PMID: 27482885 PMC link DOI: 10.1172/JCI86249
- ↑ Nekoukar, Z., Moghimi, M., & Salehifar, E. (2021). A narrative review on adverse effects of dasatinib with a focus on pharmacotherapy of dasatinib-induced pulmonary toxicities. Blood research, 56(4), 229-242. PMID: 32527740 DOI:link
- ↑ Cheng, F., Xu, Q., Li, Q., Cui, Z., Li, W., & Zeng, F. (2023). Adverse reactions after treatment with dasatinib in chronic myeloid leukemia: Characteristics, potential mechanisms, and clinical management strategies. Frontiers in Oncology, 13, 349.
- ↑ Khosla, S. (2023). Senescent cells, senolytics and tissue repair: the devil may be in the dosing. Nature Aging, 1-3. https://doi.org/10.1038/s43587-023-00365-6
- ↑ Ogrodnik, M., Miwa, S., Tchkonia, T., Tiniakos, D., Wilson, C. L., Lahat, A., ... & Jurk, D. (2017). Cellular senescence drives age-dependent hepatic steatosis. Nat Commun 8: 15691.
- ↑ Nambiar, A., Kellogg, D., Justice, J., Goros, M., Gelfond, J., Pascual, R., ... & Kirkland, J. (2023). Senolytics dasatinib and quercetin in idiopathic pulmonary fibrosis: results of a phase I, single-blind, single-center, randomized, placebo-controlled pilot trial on feasibility and tolerability. EBioMedicine, 90. PMID: 36857968 PMC10006434 DOI: 10.1016/j.ebiom.2023.104481
- ↑ Ruggiero AD, Vemuri R, Blawas M et al (2023) Long-term dasatinib plus quercetin effects on aging outcomes and inflammation in nonhuman primates: implications for senolytic clinical trial design. Geroscience. https://doi.org/10.1007/s11357-023-00830-5
- ↑ Wang, Y., Chang, J., Liu, X., Zhang, X., Zhang, S., Zhang, X., ... & Zheng, G. (2016). Discovery of piperlongumine as a potential novel lead for the development of senolytic agents. Aging (Albany NY), 8(11), 2915. PMC5191878 DOI: 10.18632/aging.101100
- ↑ Meiners, F., Secci, R., Sueto, S., Fuellen, G., & Barrantes, I. (2022). Computational identification of natural senotherapeutic compounds that mimic dasatinib based on gene expression data. bioRxiv, 2022-05. https://doi.org/10.1101/2022.05.26.492763
- ↑ Khan, N., Syed, D. N., Ahmad, N., & Mukhtar, H. (2013). Fisetin: a dietary antioxidant for health promotion. Antioxidants & redox signaling, 19(2), 151-162. PMID: 23121441 PMC link DOI: 10.1089/ars.2012.4901
- ↑ Kubina, R., Krzykawski, K., Kabała-Dzik, A., Wojtyczka, R. D., Chodurek, E., & Dziedzic, A. (2022). Fisetin, a potent anticancer flavonol exhibiting cytotoxic activity against neoplastic malignant cells and cancerous conditions: A scoping, comprehensive review. Nutrients, 14(13), 2604. PMID: 35807785 PMC link DOI: 10.3390/nu14132604
- ↑ 65.0 65.1 Yousefzadeh, M. J., Zhu, Y. I., McGowan, S. J., Angelini, L., Fuhrmann-Stroissnigg, H., Xu, M., ... & Niedernhofer, L. J. (2018). Fisetin is a senotherapeutic that extends health and lifespan. EBioMedicine, 36, 18-28. PMID: 30279143 PMC link DOI: 10.1016/j.ebiom.2018.09.015
- ↑ Elsallabi, O., Patruno, A., Pesce, M., Cataldi, A., Carradori, S., & Gallorini, M. (2022). Fisetin as a senotherapeutic agent: biopharmaceutical properties and crosstalk between cell senescence and neuroprotection. Molecules, 27(3), 738. PMID: 35164003 PMC link DOI: 10.3390/molecules27030738
- ↑ Ravula, A. R., Teegala, S. B., Kalakotla, S., Pasangulapati, J. P., Perumal, V., & Boyina, H. K. (2021). Fisetin, potential flavonoid with multifarious targets for treating neurological disorders: An updated review. European Journal of Pharmacology, 910, 174492. PMID: 34516952 DOI:link
- ↑ Shen, B., Shangguan, X., Yin, Z., Wu, S., Zhang, Q., Peng, W., ... & Chen, J. (2021). Inhibitory effect of fisetin on α-glucosidase activity: Kinetic and molecular docking studies. Molecules, 26(17), 5306. PMID: 34500738 PMC link DOI: 10.3390/molecules26175306
- ↑ Qian, X., Lin, S., Li, J., Jia, C., Luo, Y., Fan, R., ... & Chen, Y. (2023). Fisetin Ameliorates Diabetic Nephropathy-Induced Podocyte Injury by Modulating Nrf2/HO-1/GPX4 Signaling Pathway. Evidence-Based Complementary and Alternative Medicine, 2023. Article ID 9331546 https://doi.org/10.1155/2023/9331546
- ↑ Althunibat, O. Y., Al Hroob, A. M., Abukhalil, M. H., Germoush, M. O., Bin-Jumah, M., & Mahmoud, A. M. (2019). Fisetin ameliorates oxidative stress, inflammation and apoptosis in diabetic cardiomyopathy. Life sciences, 221, 83-92. PMID: 30742869 DOI:link
- ↑ Kim, H. J., Kim, S. H., & Yun, J. M. (2012). Fisetin inhibits hyperglycemia-induced proinflammatory cytokine production by epigenetic mechanisms. Evidence-Based Complementary and Alternative Medicine, 2012. PMID: 23320034 PMC link DOI: 10.1155/2012/639469
- ↑ Hasoon, D. A. A. W., Kadhim, K. A., Rahmah, A. M., & Alabbassi, M. G. (2023). Potential Effect of Fisetin in A sample of Obese Diabetic Patients in Iraq. HIV Nursing, 23(2), 277-283. https://www.hivnursing.net/index.php/hiv/article/view/1356
- ↑ 73.0 73.1 Zhu, Y., Doornebal, E. J., Pirtskhalava, T., Giorgadze, N., Wentworth, M., Fuhrmann-Stroissnigg, H., ... & Kirkland, J. L. (2017). New agents that target senescent cells: the flavone, fisetin, and the BCL-XL inhibitors, A1331852 and A1155463. Aging (Albany NY), 9(3), 955. PMID: 28273655 PMC link DOI: 10.18632/aging.101202
- ↑ Kampkötter, A., Gombitang Nkwonkam, C., Zurawski, R. F., Timpel, C., Chovolou, Y., Wätjen, W., & Kahl, R. (2007). Effects of the flavonoids kaempferol and fisetin on thermotolerance, oxidative stress and FoxO transcription factor DAF-16 in the model organism Caenorhabditis elegans. Archives of toxicology, 81, 849-858. PMID: 17551714 DOI:link
- ↑ 75.0 75.1 75.2 Park, S., Kim, B. K., & Park, S. K. (2022). Effects of Fisetin, a Plant-Derived Flavonoid, on Response to Oxidative Stress, Aging, and Age-Related Diseases in Caenorhabditis elegans. Pharmaceuticals, 15(12), 1528. PMID: 36558979 PMC link DOI: 10.3390/ph15121528
- ↑ Mbara, K. C., Devnarain, N., & Owira, P. M. (2022). Potential Role of Polyphenolic Flavonoids as Senotherapeutic Agents in Degenerative Diseases and Geroprotection. Pharmaceutical Medicine, 36(6), 331-352. PMID: 36100824 PMC link DOI: 10.1007/s40290-022-00444-w
- ↑ Carmona-Gutierrez, D., Zimmermann, A., Kainz, K., Pietrocola, F., Chen, G., Maglioni, S., ... & Madeo, F. (2019). The flavonoid 4, 4′-dimethoxychalcone promotes autophagy-dependent longevity across species. Nature communications, 10(1), 651. PMID: 30783116 PMC6381180 DOI: 10.1038/s41467-019-08555-w
- ↑ Yang, C., Wang, T., Zhao, Y., Meng, X., Ding, W., Wang, Q., ... & Deng, H. (2022). Flavonoid 4, 4′-dimethoxychalcone induced ferroptosis in cancer cells by synergistically activating Keap1/Nrf2/HMOX1 pathway and inhibiting FECH. Free Radical Biology and Medicine, 188, 14-23. PMID: 35697292 DOI: 10.1016/j.freeradbiomed.2022.06.010
- ↑ Wang, T., Yang, C., Li, Z., Li, T., Zhang, R., Zhao, Y., ... & Deng, H. (2024). Flavonoid 4, 4′-dimethoxychalcone selectively eliminates senescent cells via activating ferritinophagy. Redox Biology, 69, 103017. PMID: 38176315 DOI: 10.1016/j.redox.2023.103017
- ↑ Cherif, H., Bisson, D. G., Jarzem, P., Weber, M., Ouellet, J. A., & Haglund, L. (2019). Curcumin and o-vanillin exhibit evidence of senolytic activity in human IVD cells in vitro. Journal of Clinical Medicine, 8(4), 433. PMID: 30934902 PMC link DOI: 10.3390/jcm8040433
- ↑ Beltzig, L., Frumkina, A., Schwarzenbach, C., & Kaina, B. (2021). Cytotoxic, genotoxic and senolytic potential of native and micellar curcumin. Nutrients, 13(7), 2385. PMID: 34371895 PMC link DOI: 10.3390/nu13072385
- ↑ Li, W., He, Y., Zhang, R., Zheng, G., & Zhou, D. (2019). The curcumin analog EF24 is a novel senolytic agent. Aging (Albany NY), 11(2), 771. PMID: 30694217 PMC link DOI: 10.18632/aging.101787
- ↑ Karthika, C. et al. (2023). The Role of Curcumin as an Anti-Aging Compound. In: Rizvi, S.I. (eds) Emerging Anti-Aging Strategies. Springer, Singapore. https://doi.org/10.1007/978-981-19-7443-4_11
- ↑ Wang, L., Liu, Y., Zhou, Y., Wang, J., Tu, L., Sun, Z., ... & Luo, F. (2019). Zoledronic acid inhibits the growth of cancer stem cell derived from cervical cancer cell by attenuating their stemness phenotype and inducing apoptosis and cell cycle arrest through the Erk1/2 and Akt pathways. Journal of Experimental & Clinical Cancer Research, 38(1), 1-18. PMID: 30791957 PMCID: PMC6385443 DOI: 10.1186/s13046-019-1109-z
- ↑ Lv, J., Chen, F. K., Liu, C., Liu, P. J., Feng, Z. P., Jia, L., ... & Deng, Z. Y. (2020). Zoledronic acid inhibits thyroid cancer stemness and metastasis by repressing M2-like tumor-associated macrophages induced Wnt/β-catenin pathway. Life sciences, 256, 117925.
- ↑ Reid, I. R., Horne, A. M., Mihov, B., Stewart, A., Garratt, E., Bastin, S., & Gamble, G. D. (2020). Effects of zoledronate on cancer, cardiac events, and mortality in osteopenic older women. Journal of Bone and Mineral Research, 35(1), 20-27. PMID: 31603996 DOI: 10.1002/jbmr.3860
- ↑ Cengiz, Ö., Polat, G., Karademir, G., Tunç, O. D., Erdil, M., Tuncay, İ., & Şen, C. (2016). Effects of zoledronate on mortality and morbidity after surgical treatment of hip fractures. Advances in orthopedics, 2016.2016:3703482 PMID: 27092280 PMCID: PMC4820612 DOI: 10.1155/2016/3703482
- ↑ Samakkarnthai, P., Saul, D., Zhang, L., Aversa, Z., Doolittle, M. L., Sfeir, J., ... & Khosla, S. (2023). In vitro and in vivo effects of zoledronate on senescence and senescence-associated secretory phenotype markers. bioRxiv, 2023-02. PMID: 36865244 PMCID: PMC9980119 DOI: 10.1101/2023.02.23.529777
- ↑ Nistor, M., Pop, R., Daescu, A., Pintea, A., Socaciu, C., & Rugina, D. (2022). Anthocyanins as Key Phytochemicals Acting for the Prevention of Metabolic Diseases: An Overview. Molecules, 27(13), 4254. PMID: 35807504 PMC link DOI: 10.3390/molecules27134254
- ↑ Tena, N., Martín, J., & Asuero, A. G. (2020). State of the art of anthocyanins: Antioxidant activity, sources, bioavailability, and therapeutic effect in human health. Antioxidants, 9(5), 451. PMID: 32456252 PMC link DOI: 10.3390/antiox9050451
- ↑ Dong, Y., Wu, X., Han, L., Bian, J., He, C., El-Omar, E., ... & Wang, M. (2022). The potential roles of dietary anthocyanins in inhibiting vascular endothelial cell senescence and preventing cardiovascular diseases. Nutrients, 14(14), 2836. PMID: 35889793 PMC link DOI: 10.3390/nu14142836
- ↑ Hu, X., Yang, Y., Tang, S., Chen, Q., Zhang, M., Ma, J., ... & Yu, H. (2023). Anti-Aging Effects of Anthocyanin Extracts of Sambucus canadensis Caused by Targeting Mitochondrial-Induced Oxidative Stress. International Journal of Molecular Sciences, 24(2), 1528.
- ↑ Kim, S., Chae, J. B., Kim, D., Park, C. W., Sim, Y., Lee, H., ... & Ryu, J. H. (2023). Supramolecular Senolytics via Intracellular Oligomerization of Peptides in Response to Elevated Reactive Oxygen Species Levels in Aging Cells. Journal of the American Chemical Society. PMID: 37664981 DOI: 10.1021/jacs.3c06898
- ↑ Yu, Y., Zhou, L., Yang, Y., & Liu, Y. (2018). Cycloastragenol: An exciting novel candidate for age‑associated diseases. Experimental and therapeutic medicine, 16(3), 2175-2182. PMID: 30186456 PMC link DOI: 10.3892/etm.2018.6501
- ↑ 95.0 95.1 Zhang, Y., Gao, D., Yuan, Y., Zheng, R., Sun, M., Jia, S., & Liu, J. (2023). Cycloastragenol: A Novel Senolytic Agent That Induces Senescent Cell Apoptosis and Restores Physical Function in TBI-Aged Mice. International Journal of Molecular Sciences, 24(7), 6554. https://doi.org/10.3390/ijms24076554
- ↑ Nogueira-Recalde, U., Lorenzo-Gómez, I., Blanco, F. J., Loza, M. I., Grassi, D., Shirinsky, V., ... & Caramés, B. (2019). Fibrates as drugs with senolytic and autophagic activity for osteoarthritis therapy. EBioMedicine, 45, 588-605. PMID: 31285188 PMC link DOI: 10.1016/j.ebiom.2019.06.049
- ↑ Lu, Y., Nanayakkara, G., Sun, Y., Liu, L., Xu, K., Drummer IV, C., ... & Yang, X. (2021). Procaspase-1 patrolled to the nucleus of proatherogenic lipid LPC-activated human aortic endothelial cells induces ROS promoter CYP1B1 and strong inflammation. Redox Biology, 47, 102142. PMID: 34598017 PMC8487079 DOI: 10.1016/j.redox.2021.102142
- ↑ Murray, G. I., Taylor, M. C., McFadyen, M. C., McKay, J. A., Greenlee, W. F., Burke, M. D., & Melvin, W. T. (1997). Tumor-specific expression of cytochrome P450 CYP1B1. Cancer research, 57(14), 3026-3031. PMID: 9230218
- ↑ Yuan, B., Liu, G., Dai, Z., Wang, L., Lin, B., & Zhang, J. (2022). CYP1B1: A Novel Molecular Biomarker Predicts Molecular Subtype, Tumor Microenvironment, and Immune Response in 33 Cancers. Cancers, 14(22), 5641. PMID: 36428734 PMCID: PMC9688555 DOI: 10.3390/cancers14225641
- ↑ Ye, G., Li, J., Yu, W., Xie, Z., Zheng, G., Liu, W., ... & Shen, H. (2023). ALKBH5 facilitates CYP1B1 mRNA degradation via m6A demethylation to alleviate MSC senescence and osteoarthritis progression. Experimental & Molecular Medicine, 55(8), 1743-1756. PMID: 37524872 PMC10474288 DOI: 10.1038/s12276-023-01059-0
- ↑ Tan, H. L., Butler, P. C., Burke, M. D., & Potter, G. A. (2007). Salvestrols: a new perspective in nutritional research. Journal of Orthomolecular Medicine, 22(1), 39-47.
- ↑ DIET, R., & SHOP, N. S. (2012). Salvestrols cause cancer cell death. ICON, 2011(2010), 2010. https://www.canceractive.com/article/Salvestrols,-Protection-and-Correction
- ↑ Tan, H. L., Beresford, K., Butler, P. C., Potter, G. A., & Burke, M. D. (2007). Salvestrols-natural anticancer prodrugs in the diet. In Journal of Pharmacy and Pharmacology (Vol. 59, pp. A59-A59).
- ↑ Georg, C. S., Center, L. S., Protocol, L. T., & PDT, P. T. T. Salvestrols in Cancer and Chronic Diseases 15. December 2019 16. March 2021 Dr. Douwes informs/Prevention.
- ↑ Ferenčić, D., Gluhić, D., & Dudaš, S. (2016). Hranjiva vrijednost mandarina (Citrus reticulata Blanco, Citrus nobilis Lour). Glasnik zaštite bilja, 39(3), 46-52. https://hrcak.srce.hr/162239
- ↑ 106.0 106.1 Baar, M. P., Brandt, R. M., Putavet, D. A., Klein, J. D., Derks, K. W., Bourgeois, B. R., ... & de Keizer, P. L. (2017). Targeted apoptosis of senescent cells restores tissue homeostasis in response to chemotoxicity and aging. Cell, 169(1), 132-147. PMID: 28340339 PMC link DOI: 10.1016/j.cell.2017.02.031
- ↑ Zhang, C., Xie, Y., Chen, H., Lv, L., Yao, J., Zhang, M., ... & Liu, G. (2020). FOXO4-DRI alleviates age-related testosterone secretion insufficiency by targeting senescent Leydig cells in aged mice. Aging (Albany NY), 12(2), 1272.PMID: 31959736 PMC link DOI: 10.18632/aging.102682
- ↑ Huang, Y., He, Y., Makarcyzk, M. J., & Lin, H. (2021). Senolytic peptide FOXO4-DRI selectively removes senescent cells from in vitro expanded human chondrocytes. Frontiers in Bioengineering and Biotechnology, 9, 677576. PMID: 33996787 PMC link DOI: 10.3389/fbioe.2021.677576
- ↑ Jeon, O. H., Kim, C., Laberge, R. M., Demaria, M., Rathod, S., Vasserot, A. P., ... & Elisseeff, J. H. (2017). Local clearance of senescent cells attenuates the development of post-traumatic osteoarthritis and creates a pro-regenerative environment. Nature medicine, 23(6), 775-781.
- ↑ Hsu, B., Visich, J., Lane, N. E., Li, L., Mittal, J., An, M., ... & Dananberg, J. (2020). Safety, tolerability, pharmacokinetics, and clinical outcomes following treatment of painful knee osteoarthritis with senolytic molecule UBX0101. Osteoarthritis and Cartilage, 28, S479-S480.
- ↑ Lane, N., Hsu, B., Visich, J., Xie, B., Khan, A., & Dananberg, J. (2021). A phase 2, randomized, double-blind, placebo-controlled study of senolytic molecule UBX0101 in the treatment of painful knee osteoarthritis. Osteoarthritis and Cartilage, 29, S52-S53. DOI:10.1016/j.joca.2021.02.077
- ↑ 112.0 112.1 Al-Mansour, F., Alraddadi, A., He, B., Saleh, A., Poblocka, M., Alzahrani, W., ... & Macip, S. (2023). Characterization of the HDAC/PI3K inhibitor CUDC-907 as a novel senolytic. Aging, 15. PMID: 36988504 DOI: 10.18632/aging.204616
- ↑ Tsuruda, P., Chaney, S., Dejda, A., Dasgupta, S., Crespo-Garcia, S., Rao, S., ... & Beltran, P. (2021). UBX1325, a small molecule inhibitor of Bcl-xL, attenuates vascular dysfunction in two animal models of retinopathy. Investigative Ophthalmology & Visual Science, 62(8), 1163-1163.
- ↑ Bhisitkul, R., Klier, S., Tsuruda, P., Xie, B., Masaki, L., Bautista, J., ... & Dananberg, J. (2022). UBX1325, A Novel Senolytic Treatment for Patients with Advanced DME or wet AMD: 24-Week Results of a Phase 1 Study. Investigative Ophthalmology & Visual Science, 63(7), 4287-4287.
- ↑ Ozsvari, B., Nuttall, J. R., Sotgia, F., & Lisanti, M. P. (2018). Azithromycin and Roxithromycin define a new family of “senolytic” drugs that target senescent human fibroblasts. Aging (Albany NY), 10(11), 3294. PMID: 30428454 PMC link DOI: 10.18632/aging.101633
- ↑ Zhang, X., Dong, Y., Li, W. C., Tang, B. X., Li, J., & Zang, Y. (2021). Roxithromycin attenuates bleomycin-induced pulmonary fibrosis by targeting senescent cells. Acta Pharmacologica Sinica, 42(12), 2058-2068. PMID: 33654217 PMC link DOI: 10.1038/s41401-021-00618-3
- ↑ Robbins, R. (2018). Antibiotics as anti-inflammatories in pulmonary diseases. Southwest J Pulm Crit Care. 17(3), 97-107. doi: https://doi.org/10.13175/swjpcc104-18
- ↑ Babu, K. S., Kastelik, J., & Morjaria, J. B. (2013). Role of long term antibiotics in chronic respiratory diseases. Respiratory medicine, 107(6), 800-815.
- ↑ Mann, T. S., Larcombe, A. N., Wang, K. C., Shamsuddin, D., Landwehr, K. R., Noble, P. B., & Henry, P. J. (2022). Azithromycin inhibits mucin secretion, mucous metaplasia, airway inflammation and airways hyperresponsiveness in mice exposed to house dust mite extract. American Journal of Physiology-Lung Cellular and Molecular Physiology. 322(5), L683-L698. PMID: 35348023 DOI:link
- ↑ Garey, K. W., Alwani, A., Danziger, L. H., & Rubinstein, I. (2003). Tissue reparative effects of macrolide antibiotics in chronic inflammatory sinopulmonary diseases. Chest, 123(1), 261-265. PMID: 12527628 DOI:link
- ↑ Echeverría-Esnal, D., Martin-Ontiyuelo, C., Navarrete-Rouco, M. E., De-Antonio Cuscó, M., Ferrández, O., Horcajada, J. P., & Grau, S. (2021). Azithromycin in the treatment of COVID-19: a review. Expert review of anti-infective therapy, 19(2), 147-163. PMID: 32853038 DOI:link
- ↑ Serisier, D. J. (2013). Risks of population antimicrobial resistance associated with chronic macrolide use for inflammatory airway diseases. The Lancet Respiratory Medicine, 1(3), 262-274. PMID: 24429132 DOI:link
- ↑ 123.0 123.1 Alrashedi, M. G., Ali, A. S., Ahmed, O. A., & Ibrahim, I. M. (2022). Local Delivery of Azithromycin Nanoformulation Attenuated Acute Lung Injury in Mice. Molecules, 27(23), 8293. PMID: 36500388 PMC link DOI: 10.3390/molecules27238293
- ↑ Huynh, D. T. M., Hai, H. T., Hau, N. M., Lan, H. K., Vinh, T. P., De Tran, V., & Pham, D. T. (2023). Preparations and characterizations of effervescent granules containing azithromycin solid dispersion for children and elder: Solubility enhancement, taste-masking, and digestive acidic protection. Heliyon, 9(6). e16592 PMID: 37292293 PMC10245243 DOI: 10.1016/j.heliyon.2023.e16592
- ↑ Sadamatsu, H., Takahashi, K., Tashiro, H., Kurihara, Y., Kato, G., Uchida, M., ... & Sueoka-Aragane, N. (2020). The nonantibiotic macrolide EM900 attenuates house dust mite-induced airway inflammation in a mouse model of obesity-associated asthma. International Archives of Allergy and Immunology, 181(9), 665-674. PMID: 32599580 DOI:link
- ↑ 126.0 126.1 Cooley, J. C., Javkhlan, N., Wilson, J. A., Foster, D. G., Edelman, B. L., Ortiz, L. A., ... & Redente, E. F. (2023). Inhibition of antiapoptotic BCL-2 proteins with ABT-263 induces fibroblast apoptosis, reversing persistent pulmonary fibrosis. JCI insight, 8(3). PMID: 36752201 DOI:link
- ↑ Mohamad Anuar, N. N., Nor Hisam, N. S., Liew, S. L., & Ugusman, A. (2020). Clinical review: navitoclax as a pro-apoptotic and anti-fibrotic agent. Frontiers in Pharmacology, 1817. PMID: 33381025 PMC link DOI: 10.3389/fphar.2020.564108
- ↑ Lagares, D., Santos, A., Grasberger, P. E., Liu, F., Probst, C. K., Rahimi, R. A., ... & Tager, A. M. (2017). Targeted apoptosis of myofibroblasts with the BH3 mimetic ABT-263 reverses established fibrosis. Science Translational Medicine, 9(420), eaal3765. PMID: 29237758 PMC link DOI: 10.1126/scitranslmed.aal3765
- ↑ Takaya, K., Ishii, T., Asou, T., & Kishi, K. (2023). Navitoclax (ABT-263) rejuvenates human skin by eliminating senescent dermal fibroblasts in a mouse/human chimeric model. Rejuvenation Research. 26(1), 9-20 PMID: 36324221 DOI:link
- ↑ PMID: 36638657 DOI:org/10.1016/j.intimp.2023.109694
- ↑ Zhao, C., & Dekker, F. J. (2022). Novel Design Strategies to Enhance the Efficiency of Proteolysis Targeting Chimeras. ACS Pharmacology & Translational Science, 5(9), 710-723. PMID: 36110375 PMC link DOI: 10.1021/acsptsci.2c00089
- ↑ Burslem, G. M., & Crews, C. M. (2020). Proteolysis-targeting chimeras as therapeutics and tools for biological discovery. Cell, 181(1), 102-114. PMID: 31955850 PMC link DOI: 10.1016/j.cell.2019.11.031
- ↑ Graham, H. (2022). The mechanism of action and clinical value of PROTACs: A graphical review. Cellular Signalling, 110446. PMID: 35995302 DOI:link
- ↑ Burslem, G. M. (2023). The Future of Heterobifunctional Compounds: PROTACs and Beyond. Inducing Targeted Protein Degradation: From Chemical Biology to Drug Discovery and Clinical Applications, 273-287.
- ↑ Lee, S. J., Cho, J., Lee, B. H., Hwang, D., & Park, J. W. (2023). Design and Prediction of Aptamers Assisted by In Silico Methods. Biomedicines, 11(2), 356. https://doi.org/10.3390/biomedicines11020356
- ↑ Weng, G., Cai, X., Cao, D., Du, H., Shen, C., Deng, Y., ... & Hou, T. (2023). PROTAC-DB 2.0: an updated database of PROTACs. Nucleic Acids Research, 51(D1), D1367-D1372. PMID: 36300631 PMC link DOI: 10.1093/nar/gkac946
- ↑ Li, M., Zhi, Y., Liu, B., & Yao, Q. (2023). Advancing Strategies for Proteolysis-Targeting Chimera Design. Journal of Medicinal Chemistry. PMID: 36788245 DOI:link
- ↑ Kumar, D., & Hassan, M. I. (2022). Targeted protein degraders march towards the clinic for neurodegenerative diseases. Ageing Research Reviews, 101616. PMID: 35378298 DOI:link
- ↑ George, N., Akhtar, M. J., Balushi, K. A., Safi, S. Z., Azmi, S. N. H., & Khan, S. A. (2023). The emerging role of proteolysis targeting chimeras (PROTACs) in the treatment of Alzheimer’s disease. Medicinal Chemistry Research, 1-16.
- ↑ Xia, Y., Li, J., Wang, L., Xie, Y., Zhang, L., Han, X., ... & Liu, Y. (2023). Engineering Hierarchical Recognition‐Mediated Senolytics for Reliable Regulation of Cellular Senescence and Anti‐Atherosclerosis Therapy. Angewandte Chemie International Edition, 62(4), e202214169. PMID: 36445796 DOI:link
- ↑ 141.0 141.1 Qian, H., Zhu, M., Tan, X., Zhang, Y., Liu, X., & Yang, L. (2023). Super-enhancers and the super-enhancer reader BRD4: tumorigenic factors and therapeutic targets. Cell Death Discovery, 9(1), 470. PMID: 38135679 PMC10746725 DOI: 10.1038/s41420-023-01775-6
- ↑ Blayney, J. W., Francis, H., Rampasekova, A., Camellato, B., Mitchell, L., Stolper, R., ... & Kassouf, M. (2023). Super-enhancers include classical enhancers and facilitators to fully activate gene expression. Cell, 186(26), 5826-5839. PMID: 38101409 DOI: 10.1016/j.cell.2023.11.030
- ↑ Garcia, G., Bar‐Ziv, R., Averbukh, M., Dasgupta, N., Dutta, N., Zhang, H., ... & Higuchi‐Sanabria, R. (2023). Large‐scale genetic screens identify BET‐1 as a cytoskeleton regulator promoting actin function and life span. Aging Cell, 22(1), e13742. PMID: 36404134 PMC link DOI: 10.1111/acel.13742
- ↑ Shin, E. Y., Park, J. H., You, S. T., Lee, C. S., Won, S. Y., Park, J. J., ... & Kim, E. G. (2020). Integrin-mediated adhesions in regulation of cellular senescence. Science Advances, 6(19), eaay3909. PMID: 32494696 PMC link DOI: 10.1126/sciadv.aay3909
- ↑ Lu, J., Qian, Y., Altieri, M., Dong, H., Wang, J., Raina, K., ... & Crews, C. M. (2015). Hijacking the E3 ubiquitin ligase cereblon to efficiently target BRD4. Chemistry & biology, 22(6), 755-763. PMID: 26051217 PMC link DOI: 10.1016/j.chembiol.2015.05.009
- ↑ Guo, Z., Peng, H., & Xie, Y. (2020). BET family protein degraders poised to join the senolytic arsenal. Signal Transduction and Targeted Therapy, 5(1), 88. PMID: 32528000 PMC link DOI: 10.1038/s41392-020-0202-2
- ↑ Sato, S., Koyama, K., Ogawa, H., Murakami, K., Imakura, T., Yamashita, Y., ... & Nishioka, Y. (2023). A novel BRD4 degrader, ARV-825, attenuates lung fibrosis through senolysis and antifibrotic effect. Respiratory Investigation, 61(6), 781-792. PMID: 37741093 DOI: 10.1016/j.resinv.2023.08.003
- ↑ Zhang, M., Li, Y., Zhang, Z., Zhang, X., Wang, W., Song, X., & Zhang, D. (2023). BRD4 Protein as a Target for Lung Cancer and Hematological Cancer Therapy: A Review. Current Drug Targets, 24(14), 1079-1092. https://doi.org/10.2174/0113894501269090231012090351
- ↑ Liu, L., Guo, Y., Tian, S., Lei, I., Gao, W., Li, Z., ... & Wang, Z. (2024). Transient BRD4 degradation improves cardiac reprogramming by inhibiting macrophage/Oncostatin M induced JAK/STAT pathway. bioRxiv, 2023-12. https://doi.org/10.1101/2023.12.31.573781
- ↑ He, Y., Zhang, X., Chang, J., Kim, H. N., Zhang, P., Wang, Y., ... & Zhou, D. (2020). Using proteolysis-targeting chimera technology to reduce navitoclax platelet toxicity and improve its senolytic activity. Nature communications, 11(1), 1996. PMID: 32332723 PMC link DOI: 10.1038/s41467-020-15838-0
- ↑ Baar, M. P., Brandt, R. M., Putavet, D. A., Klein, J. D., Derks, K. W., Bourgeois, B. R., ... & de Keizer, P. L. (2017). Targeted apoptosis of senescent cells restores tissue homeostasis in response to chemotoxicity and aging. Cell, 169(1), 132-147.
- ↑ Khan, S., Zhang, X., Lv, D., Zhang, Q., He, Y., Zhang, P., ... & Zhou, D. (2019). A selective BCL-XL PROTAC degrader achieves safe and potent antitumor activity. Nature medicine, 25(12), 1938-1947. PMID: 31792461 PMC link DOI: 10.1038/s41591-019-0668-z
- ↑ Acunzo, J., Katsogiannou, M., & Rocchi, P. (2012). Small heat shock proteins HSP27 (HspB1), αB-crystallin (HspB5) and HSP22 (HspB8) as regulators of cell death. The international journal of biochemistry & cell biology, 44(10), 1622-1631. PMID: 22521623 DOI:link
- ↑ Zhang, Y., Li, C., Meng, H., Guo, D., Zhang, Q., Lu, W., ... & Tu, P. (2018). BYD ameliorates oxidative stress-induced myocardial apoptosis in heart failure post-acute myocardial infarction via the P38 MAPK-CRYAB signaling pathway. Frontiers in Physiology, 9, 505. PMID: 29867551 PMC link DOI: 10.3389/fphys.2018.00505
- ↑ Delbecq, S. P., & Klevit, R. E. (2019). HSPB5 engages multiple states of a destabilized client to enhance chaperone activity in a stress-dependent manner. Journal of Biological Chemistry, 294(9), 3261-3270. PMID: 30567736 PMC link DOI: 10.1074/jbc.RA118.003156
- ↑ Chebotareva, N. A., Roman, S. G., Borzova, V. A., Eronina, T. B., Mikhaylova, V. V., & Kurganov, B. I. (2020). Chaperone-like activity of HSPB5: The effects of quaternary structure dynamics and crowding. International Journal of Molecular Sciences, 21(14), 4940. PMID: 32668633 PMC link DOI: 10.3390/ijms21144940
- ↑ Dimauro, I., & Caporossi, D. (2022). Alpha B-Crystallin in Muscle Disease Prevention: The Role of Physical Activity. Molecules, 27(3), 1147. PMID: 35164412 PMC link DOI: 10.3390/molecules27031147
- ↑ Rashidieh, B., Bain, A. L., Tria, S. M., Sharma, S., Stewart, C. A., Simmons, J. L., ... & Khanna, K. K. (2023). Alpha-B-Crystallin overexpression is sufficient to promote tumorigenesis and metastasis in mice. Experimental Hematology & Oncology, 12(1), 4. PMID: 36624493 PMC link DOI: 10.1186/s40164-022-00365-z
- ↑ Hayashi, J., & Carver, J. A. (2020). The multifaceted nature of αB-crystallin. Cell Stress and Chaperones, 25, 639-654. PMID: 32383140 PMC link DOI: 10.1007/s12192-020-01098-w
- ↑ Limbad, C., Doi, R., McGirr, J., Ciotlos, S., Perez, K., Clayton, Z. S., ... & Melov, S. (2022). Senolysis induced by 25-hydroxycholesterol targets CRYAB in multiple cell types. Iscience, 25(2), 103848. PMID: 35198901 PMC link DOI: 10.1016/j.isci.2022.103848
- ↑ Sugiura, H., Koarai, A., Ichikawa, T., Minakata, Y., Matsunaga, K., Hirano, T., ... & Ichinose, M. (2012). Increased 25‐hydroxycholesterol concentrations in the lungs of patients with chronic obstructive pulmonary disease. Respirology, 17(3), 533-540. PMID: 22295989 DOI:link
- ↑ Ichikawa, T., Sugiura, H., Koarai, A., Kikuchi, T., Hiramatsu, M., Kawabata, H., ... & Ichinose, M. (2013). 25-hydroxycholesterol promotes fibroblast-mediated tissue remodeling through NF-κB dependent pathway. Experimental cell research, 319(8), 1176-1186. PMID: 23485764 DOI:link
- ↑ Smer-Barreto, V., Quintanilla, A., Elliot, R. J., Dawson, J. C., Sun, J., Carragher, N., ... & Oyarzun, D. A. (2022). Discovery of new senolytics using machine learning. Nat Commun 14, 3445 (2023). https://doi.org/10.1038/s41467-023-39120-1, bioRxiv, 2022-04. https://doi.org/10.1101/2022.04.26.489505
- ↑ 164.0 164.1 Doan, L., Paine, P., Tran, C., Parsons, B., Hiller, A., Joshua, I., ... & Quarta, M. (2020). Targeted senolytic prodrug is well tolerated and results in amelioration of frailty, muscle regeneration and cognitive functions in geriatric mice. https://doi.org/10.21203/rs.3.rs-92962/v1
- ↑ Cai, Y., Zhou, H., Zhu, Y., Sun, Q., Ji, Y., Xue, A., ... & Deng, H. (2020). Elimination of senescent cells by β-galactosidase-targeted prodrug attenuates inflammation and restores physical function in aged mice. Cell research, 30(7), 574-589. PMID: 32341413 PMC link DOI: 10.1038/s41422-020-0314-9
- ↑ Morsli, S., Doherty, G. J., & Muñoz-Espín, D. (2022). Activatable senoprobes and senolytics: Novel strategies to detect and target senescent cells. Mechanisms of Ageing and Development, 202, 111618. PMID: 34990647 DOI:link
- ↑ 167.0 167.1 Shi, D., Liu, W., Gao, Y., Li, X., Huang, Y., Li, X., ... & Li, J. (2023). Photoactivatable senolysis with single-cell resolution delays aging. Nature Aging, 1-16. DOI:10.1038/s43587-023-00360-x
- ↑ 168.0 168.1 Takaya K, Asou T, Kishi K (2022). Selective Elimination of Senescent Fibroblasts by Targeting the Cell Surface Protein ACKR3. International journal of molecular sciences. 23(12): 6531. PMID 35742971 PMC link doi:10.3390/ijms23126531
- ↑ Liberale, L., Ministrini, S., Carbone, F., Camici, G. G., & Montecucco, F. (2021). Cytokines as therapeutic targets for cardio-and cerebrovascular diseases. Basic Research in Cardiology, 116, 1-26.PMID: 33770265 PMC link DOI: 10.1007/s00395-021-00863-x
- ↑ Takaya, K., Asou, T., & Kishi, K. (2022). Identification of Apolipoprotein D as a dermal fibroblast marker of human aging for development of skin rejuvenation therapy. Rejuvenation Research, (ja).
- ↑ A single injection resulted in 90% reduction in solid tumours after 48 hours.
- ↑ Garcia H. et al., & Lewis J.D. (2023). SYSTEMIC SENOLYSIS USING A GENETIC MEDICINE IMPROVES HEALTHSPAN IN NATURALLY AGED MICE. Abstracts of 13TH INTERNATIONAL CONFERENCE ON FRAILTY & SARCOPENIA RESEARCH (ICFSR)
- ↑ Gabandé‐Rodríguez, E., Pfeiffer, M., & Mittelbrunn, M. (2023). Immuno (T) herapy for age‐related diseases. EMBO Molecular Medicine, 15(1), e16301. PMID: 36373340 PMC link DOI: 10.15252/emmm.202216301
- ↑ Delaney, J. A., Olson, N. C., Sitlani, C. M., Fohner, A. E., Huber, S. A., Landay, A. L., ... & Doyle, M. F. (2021). Natural killer cells, gamma delta T cells and classical monocytes are associated with systolic blood pressure in the multi-ethnic study of atherosclerosis (MESA). BMC Cardiovascular Disorders, 21, 1-9. PMID: 33482725 PMC7821496 DOI: 10.1186/s12872-021-01857-2
- ↑ Lee, Y. K., Suh, E., Oh, H., Haam, J. H., & Kim, Y. S. (2024). Decreased natural killer cell activity as a potential predictor of hypertensive incidence. Frontiers in Immunology, 15, 1376421. PMID: 38715619 PMC11074345 DOI: 10.3389/fimmu.2024.1376421
- ↑ Cho, A. R., Suh, E., Oh, H., Cho, B. H., Gil, M., & Lee, Y. K. (2023). Low Muscle and High Fat Percentages Are Associated with Low Natural Killer Cell Activity: A Cross-Sectional Study. International Journal of Molecular Sciences, 24(15), 12505. PMID: 37569879 PMC10419953 DOI: 10.3390/ijms241512505
- ↑ Ogata, K., Yokose, N., Tamura, H., An, E., Nakamura, K., Dan, K., & Nomura, T. (1997). Natural killer cells in the late decades of human life. Clinical Immunology and Immunopathology, 84(3), 269-275. PMID: 9281385 DOI: 10.1006/clin.1997.4401
- ↑ Ogata, K., An, E., Shioi, Y., Nakamura, K., Luo, S., Yokose, N., ... & Dan, K. (2001). Association between natural killer cell activity and infection in immunologically normal elderly people. Clinical & Experimental Immunology, 124(3), 392-397. PMID: 11472399 PMC1906081 DOI: 10.1046/j.1365-2249.2001.01571.x
- ↑ 179.0 179.1 Huang, Y., & Liu, T. (2020). Step further towards targeted senolytic therapy: therapeutic potential of uPAR-CAR T cells for senescence-related diseases. Signal Transduction and Targeted Therapy, 5(1), 155. PMID: 32792494 PMC7426266 DOI: 10.1038/s41392-020-00268-7
- ↑ Amor, C., Feucht, J., Leibold, J., Ho, Y. J., Zhu, C., Alonso-Curbelo, D., ... & Lowe, S. W. (2020). Senolytic CAR T cells reverse senescence-associated pathologies. Nature, 583(7814), 127-132. PMID: 32555459 PMC link DOI: 10.1038/s41586-020-2403-9
- ↑ Aghajanian, H., Kimura, T., Rurik, J. G., Hancock, A. S., Leibowitz, M. S., Li, L., ... & Epstein, J. A. (2019). Targeting cardiac fibrosis with engineered T cells. Nature, 573(7774), 430-433. PMID: 31511695 PMC link DOI: 10.1038/s41586-019-1546-z
- ↑ Ferrer-Curriu, G., Soler-Botija, C., Charvatova, S., Motais, B., Roura, S., Galvez-Monton, C., ... & Genís, A. B. (2023). Preclinical scenario of targeting myocardial fibrosis with chimeric antigen receptor (CAR) immunotherapy. Biomedicine & Pharmacotherapy, 158, 114061. PMID: 36495661 DOI:link
- ↑ Yang, D., Sun, B., Li, S., Wei, W., Liu, X., Cui, X., ... & Zhao, X. (2023). NKG2D-CAR T cells eliminate senescent cells in aged mice and nonhuman primates. Science Translational Medicine, 15(709), eadd1951. PMID: 37585504 DOI: 10.1126/scitranslmed.add1951
- ↑ Friedman, S. L. (2022). Fighting cardiac fibrosis with CAR T cells. New England Journal of Medicine, 386(16), 1576-1578. PMID: 35443114 DOI:link
- ↑ Rurik, J. G., Tombácz, I., Yadegari, A., Méndez Fernández, P. O., Shewale, S. V., Li, L., ... & Epstein, J. A. (2022). CAR T cells produced in vivo to treat cardiac injury. Science, 375(6576), 91-96. PMID: 34990237 DOI:link
- ↑ Yang, L., Gong, L., Wang, P., Zhao, X., Zhao, F., Zhang, Z., ... & Huang, W. (2022). Recent Advances in Lipid Nanoparticles for Delivery of mRNA. Pharmaceutics, 14(12), 2682. PMID: 36559175 PMC link DOI: 10.3390/pharmaceutics14122682
- ↑ Ye, B., Hu, Y., Zhang, M., & Huang, H. (2022). Research advance in lipid nanoparticle-mRNA delivery system and its application in CAR-T cell therapy. Zhejiang da xue xue bao. Yi xue ban= Journal of Zhejiang University. Medical Sciences, 51(2), 185-191. PMID: 36161298 PMC link DOI: 10.3724/zdxbyxb-2022-0047
- ↑ Amor, C., Fernández-Maestre, I., Chowdhury, S. et al. (2024). Prophylactic and long-lasting efficacy of senolytic CAR T cells against age-related metabolic dysfunction. Nat Aging https://doi.org/10.1038/s43587-023-00560-5 PMID: 37841853 PMC10571605 DOI: 10.21203/rs.3.rs-3385749/v1
- ↑ Qi, C., & Liu, Q. (2023). Natural killer cells in aging and age-related diseases. Neurobiology of Disease, 183, 106156. PMID: 37209924 DOI: 10.1016/j.nbd.2023.106156
- ↑ Bexte, T., & Ullrich, E. (2024). Empowering virus-free CAR immune cell therapies. Molecular Therapy. 32(6), P1609-1611 PMID 38795701 PMC 11184381 doi:10.1016/j.ymthe.2024.05.023
- ↑ 191.0 191.1 Suda, M., Shimizu, I., Katsuumi, G., Hsiao, C. L., Yoshida, Y., Matsumoto, N., ... & Minamino, T. (2022). Glycoprotein nonmetastatic melanoma protein B regulates lysosomal integrity and lifespan of senescent cells. Scientific reports, 12(1), 1-14. PMID: 35444208 PMC link DOI: 10.1038/s41598-022-10522-3
- ↑ 192.0 192.1 192.2 Suda, M., Shimizu, I., Katsuumi, G., Yoshida, Y., Hayashi, Y., Ikegami, R., ... & Minamino, T. (2021). Senolytic vaccination improves normal and pathological age-related phenotypes and increases lifespan in progeroid mice. Nature Aging, 1(12), 1117-1126. https://doi.org/10.1038/s43587-021-00151-2
- ↑ Mendelsohn, A. R., & Larrick, J. W. (2022). Antiaging vaccines targeting senescent cells. Rejuvenation Research, 25(1), 39-45. https://doi.org/10.1089/rej.2022.0008
- ↑ Zhang, L., Pitcher, L. E., Prahalad, V., Niedernhofer, L. J., & Robbins, P. D. (2022). Targeting cellular senescence with senotherapeutics: senolytics and senomorphics. The FEBS Journal. PMID: 35015337 DOI:link
- ↑ Wang, E. (1995). Senescent human fibroblasts resist programmed cell death, and failure to suppress bcl 2 is involved. Cancer research, 55(11), 2284-2292. PMID: 7757977
- ↑ Salminen, A., Ojala, J., & Kaarniranta, K. (2011). Apoptosis and aging: increased resistance to apoptosis enhances the aging process. Cellular and molecular life sciences, 68, 1021-1031. PMID: 21116678 DOI:link
- ↑ Jochems, F., Thijssen, B., De Conti, G., Jansen, R., Pogacar, Z., Groot, K., ... & Bernards, R. (2021). The Cancer SENESCopedia: A delineation of cancer cell senescence. Cell reports, 36(4), 109441.
- ↑ Gonzalez-Gualda, E., Baker, A. G., Fruk, L., & Munoz-Espin, D. (2020). A guide to assessing cellular senescencein in vitro and in vivo. FEBS JOURNAL. 288(1), 56-80 PMID: 32961620 DOI:link
- ↑ Saul, D., Kosinsky, R. L., Atkinson, E. J., Doolittle, M. L., Zhang, X., LeBrasseur, N. K., ... & Khosla, S. (2022). A new gene set identifies senescent cells and predicts senescence-associated pathways across tissues. Nature communications, 13(1), 4827. PMID: 35974106 PMC link DOI: 10.1038/s41467-022-32552-1
- ↑ Cohn, R. L., Gasek, N. S., Kuchel, G. A., & Xu, M. (2023). The heterogeneity of cellular senescence: Insights at the single-cell level. Trends in cell biology, 33(1), 9-17. PMID: 35599179 PMCID: PMC9812642 link] DOI: 10.1016/j.tcb.2022.04.011
- ↑ Hu, L., Li, H., Zi, M., Li, W., Liu, J., Yang, Y., ... & He, Y. (2022). Why senescent cells are resistant to apoptosis: An insight for senolytic development. Frontiers in Cell and Developmental Biology, 10. PMID: 35252191 PMC link DOI: 10.3389/fcell.2022.822816
- ↑ L'Hôte, V., Mann, C., & Thuret, J. Y. (2022). From the divergence of senescent cell fates to mechanisms and selectivity of senolytic drugs. Open Biology, 12(9), 220171. PMID: 36128715 PMC link DOI: 10.1098/rsob.220171
- ↑ Salminen, A., Ojala, J., & Kaarniranta, K. (2011). Apoptosis and aging: increased resistance to apoptosis enhances the aging process. Cellular and molecular life sciences, 68, 1021-1031. PMID: 21116678 DOI: 10.1007/s00018-010-0597-y
- ↑ 204.0 204.1 Soto-Gamez, A., Quax, W. J., & Demaria, M. (2019). Regulation of survival networks in senescent cells: from mechanisms to interventions. Journal of molecular biology, 431(15), 2629-2643. PMID:31153901 DOI: 10.1016/j.jmb.2019.05.036
- ↑ Kesavardhana, S., Malireddi, R. S., & Kanneganti, T. D. (2020). Caspases in cell death, inflammation, and pyroptosis. Annual review of immunology, 38, 567-595. PMID: 32017655 PMCID: PMC7190443 DOI: 10.1146/annurev-immunol-073119-095439
- ↑ Deveraux, Q. L., Schendel, S. L., & Reed, J. C. (2001). Antiapoptotic proteins: the bcl-2 and inhibitor of apoptosis protein families. Cardiology Clinics, 19(1), 57-74. PMID: 11787814 DOI: 10.1016/s0733-8651(05)70195-8
- ↑ Ola, M. S., Nawaz, M., & Ahsan, H. (2011). Role of Bcl-2 family proteins and caspases in the regulation of apoptosis. Molecular and cellular biochemistry, 351, 41-58. PMID: 21210296 DOI: 10.1007/s11010-010-0709-x
- ↑ Silke, J., & Vucic, D. (2014). IAP family of cell death and signaling regulators. Methods in enzymology, 545, 35-65. PMID: 25065885 DOI: 10.1016/B978-0-12-801430-1.00002-0
- ↑ Hrdinka, M., & Yabal, M. (2019). Inhibitor of apoptosis proteins in human health and disease. Genes & Immunity, 20(8), 641-650. PMID: 31110240 DOI: 10.1038/s41435-019-0078-8
- ↑ Du, C., Fang, M., Li, Y., Li, L., & Wang, X. (2000). Smac, a mitochondrial protein that promotes cytochrome c–dependent caspase activation by eliminating IAP inhibition. Cell, 102(1), 33-42. PMID: 10929711 DOI: 10.1016/s0092-8674(00)00008-8
- ↑ Chakraborty, A., Bose, R., & Bose, K. (2022). Unraveling the Dichotomy of Enigmatic Serine Protease HtrA2. Frontiers in Molecular Biosciences, 66. PMID: 35187085 PMCID: PMC8850690 DOI: 10.3389/fmolb.2022.824846
- ↑ Silke, J., & Meier, P. (2013). Inhibitor of apoptosis (IAP) proteins–modulators of cell death and inflammation. Cold Spring Harbor perspectives in biology, 5(2), a008730. PMID: 23378585 PMCID: PMC3552501 DOI: 10.1101/cshperspect.a008730
- ↑ Eckelman, B. P., Drag, M., Snipas, S. J., & Salvesen, G. S. (2008). The mechanism of peptide-binding specificity of IAP BIR domains. Cell Death & Differentiation, 15(5), 920-928. PMID: 18239672 DOI: 10.1038/cdd.2008.6
- ↑ Hunkeler, M., Jin, C. Y., & Fischer, E. S. (2023). Structures of BIRC6-client complexes provide a mechanism of Smac-mediated release of caspases. Science, 379(6637), 1105-1111 DOI: 10.1126/science.ade57
- ↑ Ehrmann, J. F., Grabarczyk, D. B., Heinke, M., Deszcz, L., Kurzbauer, R., Hudecz, O., ... & Clausen, T. (2023). Structural basis for regulation of apoptosis and autophagy by the BIRC6/SMAC complex. Science, 379(6637), 1117-1123 DOI: 10.1126/science.ade88

This article is currently a draft.
Material may not yet be complete, information may presently be omitted, and certain parts of the content may be subject to radical, rapid alteration. More information pertaining to this may be available on the talk page.