Extracellular vesicles
Extracellular vesicles (EVs) is a collective term covering various subtypes of cell-released membranous structures that can be found in various body fluids, including blood plasma, urine, saliva, amniotic fluid, breast milk, and fluid that accumulates in pleural ascites. Released by almost all cell types, they play an important role in cellular communication transporting biological molecules between cells, as they carry bioactive proteins, lipids, and nucleic acids as part of their functional cargo, transporting biological molecules between cells.[1]
EVs acts as one of the SASP (senescence-associated secretory phenotype) factors, which enhance the proliferation of cancer cells, paracrine senescence, and chromosomal instability.[2][3] Among the hallmarks of senescence, the SASP, especially SASP-related extracellular vesicle (EV) signalling, plays the leading role in aging transmission via paracrine and endocrine mechanisms.[4][5][6]
EV classification and composition
EVs encompass various types of vesicles,[7][4] including:
- Exosomes (30–100 nm, the smallest extracellular vesicle) formation and release occur through the endosomal pathway and into the extracellular medium after fusion with the plasma membrane. Its content corresponds to that existing in the endosomal compartment.
- Ectosomes (100–350 nm) are vesicles found everywhere in organisms and released from the plasma membrane. Their function is analogous to exosomes.
- Microvesicles (MVs; formerly called microparticles or MPs) have a size from 100 nm–1 µm. They are secreted outside the cell by the process of evagination or sprouting of the plasma membrane, which involves: (a) relocation of phospholipids in the outer membrane so that the phosphatidylserine (PS), generally located on the inner side of the membrane, is exposed on the surface of the vesicle, (b) rearrangement of the cytoskeleton, (c) generation of the curvature of the membrane, and (d) liberation of the vesicle.
- Apoptotic bodies (1–5 µm) are released as vesicles after cellular apoptosis, followed by increased membrane permeability, DNA fragmentation, and changes in mitochondrial membrane potential. Apoptotic bodies also expose PS on their surface and contain cellular organelles and genetic material.
- Exophers are the 3.5–4-μm large type of EV, which contain damaged mitochondria and protein aggregates
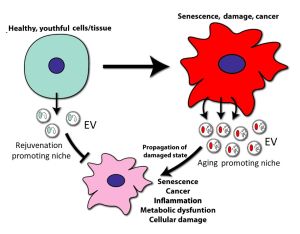
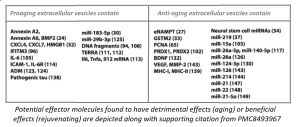
The role of extracellular vesicles in cellular senescence
Extracellular vesicles production is upregulated in senescent cells up to 50-fold,[9] with senescent-induced changes to their cargo (e.g., of proteins, miRNAs, and mRNAs).[10][11][12][4]
It was found that senescent human fibroblast cells can induce a bystander effect, spreading senescence in intact neighboring fibroblasts in vitro,[13] and that small extracellular vesicles from senescent cells are responsible for mediating paracrine senescence to nearby cells.[14][15][4][16] The outcome of their production can be either beneficial or detrimental, depending on the context.[17]
Diagnostic Roles of EV in Aging-Related Diseases
Urinal EVs have a major role in the prediction of the response to therapy in urogenital system diseases.[19] Indeed, the identification of specific biomarkers, including protein, lipids, and miRNAs, within urinary EVs unveils the prognosis of the prostate, bladder, and renal cancers [20] EVs not only serve as biomarker reservoir but also as messengers to and from kidney tubular cells.[21]
Therapeutic Roles of EV
Mesenchymal stem cell‐derived extracellular vesicles
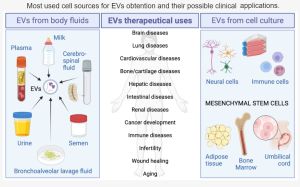
Mesenchymal stem/stromal cells (MSCs) are under clinical development for the treatment of numerous disease indications. There is growing interest surrounding the therapeutic application of purified and concentrated regenerative factors secreted by MSCs. Mesenchymal stem cells (MSCs)-derived EVs have been observed to implement the same therapeutic effects as MSCs with minimal adverse effects and could be used as an alternative treatment method to MSC-based therapy.[23][24][25][26][27] [28] Donor variance in age, sex, and other genetic differences creates significant variability in the therapeutic potency of MSCs and their secreted EVs.[29]
In contrast to stem cells, EVs like exosomes cannot self-replicate, eliminating concerns about potential tumour formation after stem cell transplantation. Exosomes are also stable enough for long-term frozen storage and storage at room temperature after lyophilization. Their small size further allows sterilization by filtration.[30][31] In addition, exosomes can be administered by several routes; for example, nebulized or lyophilized lung stem cell-derived exosomes can be administrated by inhalation to treat lung diseases.[22]
Parabiosis experiments in mice demonstrated that a young environment could partially rejuvenate multiple tissues of old organisms and it was applied to demonstrate a lifespan-enhancing effect of young blood.[32] EVs isolated from young (4-to-12-month-old) mouse plasma injected into 26-month-old female mice once a week until sacrifice led to increase of 10.2% and of 15.8% in median and maximal lifespan, respectively, in mice receiving the treatment vs. vehicle-treated mice of the same age.[33] So, it was suggested that the beneficial effects of young blood may be recapitulated by EVs transfusion.[33]
Сonditioned media (CM) from young bone marrow-derived mesenchymal stem cells (BM-MSCs) culture rescued the function of aged, senescent stem cells and senescent murine embryonic fibroblasts (MEFs) in culture, whereas CM from young BM-MSCs depleted of extracellular vesicles was unable to reduce the percentage of senescent aged BM-MSCs. Moreover, the senotherapeutic activity of CM co-purified with extracellular vesicles that were released by young, but not old MSCs and muscle-derived stem/progenitor cells (MDSPC)s. Treatment with EVs isolated from human embryonic stem cell-derived MSCs (hESC-MSC) was capable of significantly reducing the expression of markers of senescence in cultured senescent fibroblasts as well as naturally aged wild-type mice, and improving measures of healthspan in vivo. These results identified EVs as key factors released by young, functional stem cells that can rescue cellular senescence and stem cell dysfunction in culture and reduce senescent cell burden in vivo.[34][35] Small EVs (sEVs) derived from multiple stem cells, such as exosomes, have demonstrated their capacity to promote tissue regeneration after several types of damage.[36][37] Compared to stem cells, sEVs are more stable, have no risk of aneuploidy, have a lower chance of immune rejection, and can provide an alternative therapy for various diseases. It has been shown that sEVs can exert proregenerative effects in tissues of old mice and decrease senescence-related damage.[38][39][40]
Old animals treated with small extracellular vesicles derived from adipose mesenchymal stem cells (ADSCs) of young animals, revealed an improvement in several parameters usually altered with aging, such as motor coordination, grip strength, fatigue resistance, fur regeneration, and renal function, as well as an important decrease in frailty.[41] ADSC-sEVs induced proregenerative effects and a decrease in oxidative stress, inflammation, and senescence markers in muscle and kidney. Moreover, predicted epigenetic age was lower in tissues of old mice treated with ADSC-sEVs and their metabolome changed to a youth-like pattern.[41] Analysis of miRNAs in sEVs from young ADSC cultures showed several differentially expressed miRNAs when compared to sEVs from old ADSC cultures (9 up-regulated and 1 down-regulated) and from plasma of aged mice (25 up-regulated and 4 down-regulated. Six miRNAs were outlined as plausible biologically relevant, i.e., that can be considered relevant because these miRNAs are the ones that share their nucleotide sequence among several species, including humans, as many features of the aging process are highly conserved across species.[42][41]
sEVs from young cells ameliorate senescence in a variety of tissues in old mice.[43] It was identified that sEVs have intrinsic glutathione-S-transferase activity partially due to the high levels of expression of the glutathione-related protein (GSTM2).[43] Transfection of recombinant GSTM2 into sEVs derived from old fibroblasts restores their antioxidant capacity. sEVs increase the levels of reduced glutathione and decrease oxidative stress and lipid peroxidation both in vivo and in vitro.[43]
In experiments with exosomes, modified with miRNAs it was found that elevated miR-26a enhanced axonal growth in hippocampal neurons and axonal regeneration in the peripheral nervous system, and that exosomes with overexpressed miR-26a could activate the mammalian target of rapamycin (mTOR) pathway to enhance axonal growth and renewal in the nervous system, thus promoting neurogenesis.[44][45]
Extracellular Vesicles from Urine and Urine-derived Stem Cell
The presence of EVs in normal urine was first documented by electron microscopy images of pelletable material (100,000 × g ultracentrifugation) in 1986.[46] Urine can be collected non-invasively and repeatedly and then used to prepare small portions of extracellular vesicles that can be frozen and pooled later.[47] To isolate urinary extracellular vesicles, one can, for example, use an easily adoptable protocol of polyethylene glycol-based isolation.[48] Precipitation by polyethylene glycol (PEG) is fast, cost-effective, and has the highest extracellular vesicles recovery rate but is associated with low EV purity, aggregation, and retention of the polymer.[49][50][51]
Extruded cell nanovesicles
The clinical translation of EVs is constrained by the poor yield of EVs. Extrusion has recently become an effective technique for producing a large scale of nanovesicles (NVs). Proteomics and RNA sequencing data revealed that NVs resemble MSCs more closely than EVs. Intravenous delivery of MSC NVs improved heart repair and cardiac function in a mouse model of myocardial infarction.[52]
Undifferentiated iPSCs as a source for therapeutic EV production
Production of MSC EVs is currently hampered by donor-specific characteristics and limited ex vivo expansion capabilities before decreased potency, thus restricting their potential as a scalable and reproducible therapeutic.[53] Induced pluripotent stem cells (iPSCs) represent a self-renewing source for obtaining differentiated iPSC-derived MSCs (iMSCs), circumventing both scalability and donor variability concerns for therapeutic EV production. Compared with tissue-derived MSC, iMSC closely resemble their primary counterparts in morphology, immunophenotype, and three-lineage differentiation capacity while showing stronger regeneration ability in animal disease models. Moreover, iPSC can be passed down indefinitely so that the sources of iMSC can be endless and iMSC induced from a single iPSC cell or clone are theoretically more homogeneous.[54][55]
In experiments utilizing undifferentiated iPSC EVs as a control, it was found that their vascularization bioactivity was similar and their anti-inflammatory bioactivity was superior to donor-matched iMSC EVs in cell-based assays. Combined with the lack of additional differentiation steps required for iMSC generation, these results support the use of undifferentiated certain proven iPSC lines as a source for therapeutic EV production with respect to both scalability and efficacy.[56]
Exosomes as the delivery agents for reprogramming
Commonly, viruses are used as delivery agents of DNA/RNA molecules for reprogramming. However, they are problematic in that certain viruses (retrovirus, lentivirus) are integrating and for others (AAVs) there exists a significant number of individuals with immunity against them. In contrast to viruses, exosomes are not recognized by the host immune system and they are naturally adapted to delivering multiple molecules. Moreover, they may display selective transmission of the genetic information to the tissue/cell, probably on account of their high expression levels of adhesion molecules, such as integrins and tetraspanins, with their potential capability to select target. For instance, C166-derived exosomes are an effective delivery agent in that they appear to be selective for cardiac fibroblasts in vivo. C166-derived exosomes are readily taken up by cardiac fibroblasts while rarely internalized by cardiomyocytes or endothelial cells.[57] This made it possible to successfully reprogram in situ adult cardiac fibroblasts into neonatal fibroblasts which tend to produce more collagen, nestin and smooth muscle actin than their adult counterparts and being associated with improved healing outcomes due to their regenerative capacity.[58]
Strategies for Engineering of Extracellular Vesicles
Preconditioning of the cells that secrete the EVs
EVs are an important component of cell communication and they carry a cargo that is similar to their parent cell. Cells respond differently based on their microenvironment, and so it is expected that the therapeutic potential of these vesicles can be modulated by the enrichment of their parent cell microenvironment. There are various ways to increase the therapeutic efficacy of extracellular vesicles through different methods for MSC preconditioning, including chemical induction, culture conditions, and genetic modifications.[60][61][62]
In particular MSCs or their exosomes can be more efficacious after being pretreated with non-coding RNAs, growth factors, anti-inflammatory or inflammatory mediators, and hypoxia. Similarly, viral vector-mediated overexpression of particular genes can augment the protective effects of MSCs on goal of therapy.[63]
EVs secreted by hypoxic preconditioned cells have been shown to promote the proliferation of a variety of cell types.[64][65][66]
Further reading
- Syromiatnikova, V., Prokopeva, A., & Gomzikova, M. (2022). Methods of the Large-Scale Production of Extracellular Vesicles. International Journal of Molecular Sciences, 23(18), 10522. DOI: 10.3390/ijms231810522
- Son, J. P., Kim, E. H., Shin, E. K., Kim, D. H., Sung, J. H., Oh, M. J., ... & Bang, O. Y. (2023). Mesenchymal Stem Cell-Extracellular Vesicle Therapy for Stroke: Scalable Production and Imaging Biomarker Studies. Stem Cells Translational Medicine, szad034. PMID: 37311045 DOI: 10.1093/stcltm/szad034
- Ju, Y., Hu, Y., Yang, P., Xie, X., & Fang, B. (2022). Extracellular vesicle-loaded hydrogels for tissue repair and regeneration. Materials Today Bio, 100522. PMID: 36593913 PMC: 9803958 DOI: 10.1016/j.mtbio.2022.100522
- Erdbrügger, U., Blijdorp, C. J., Bijnsdorp, I. V., Borràs, F. E., Burger, D., Bussolati, B., ... & Martens‐Uzunova, E. S. (2021). Urinary extracellular vesicles: A position paper by the Urine Task Force of the International Society for Extracellular Vesicles. Journal of Extracellular Vesicles, 10(7). PMID: 34035881 PMC:8138533 DOI: 10.1002/jev2.12093
- Bajo-Santos, C., Priedols, M., Kaukis, P., Paidere, G., Gerulis-Bergmanis, R., Mozolevskis, G., ... & Rimsa, R. (2023). Extracellular Vesicles Isolation from Large Volume Samples Using a Polydimethylsiloxane-Free Microfluidic Device. International Journal of Molecular Sciences, 24(9), 7971. PMID: 37175677 PMC:10178709 DOI: 10.3390/ijms24097971
- Grigorian Shamagian, L., Rogers, R. G., Luther, K., Angert, D., Echavez, A., Liu, W., ... & Marbán, E. (2023). Rejuvenating effects of young extracellular vesicles in aged rats and in cellular models of human senescence. Scientific Reports, 13(1), 12240. PMID: 37507448 PMC10382547 DOI: 10.1038/s41598-023-39370-5
- Yu, L., Wen, H., Liu, C., Wang, C., Yu, H., Zhang, K., ... & Liu, N. (2023). Embryonic stem cell-derived extracellular vesicles rejuvenate senescent cells and antagonize aging in mice. Bioactive Materials, 29, 85-97. PMID: 37449253 PMC:10336196 DOI: 10.1016/j.bioactmat.2023.06.011
- Misawa, T., Hitomi, K., Miyata, K., Tanaka, Y., Fujii, R., Chiba, M., ... & Takahashi, A. (2023). Identification of Novel Senescent Markers in Small Extracellular Vesicles. International journal of molecular sciences, 24(3), 2421. PMID: 36768745 PMC:link DOI: 10.3390/ijms24032421
References
- ↑ Mathieu, M., Martin-Jaular, L., Lavieu, G., & Théry, C. (2019). Specificities of secretion and uptake of exosomes and other extracellular vesicles for cell-to-cell communication. Nature cell biology, 21(1), 9-17. PMID: 30602770 DOI: [https://doi.org/10.1038/s41556-018-0250-9
- ↑ Tanaka, Y., & Takahashi, A. (2021). Senescence-associated extracellular vesicle release plays a role in senescence-associated secretory phenotype (SASP) in age-associated diseases. The Journal of Biochemistry, 169(2), 147-153. PMID: 33002139 DOI: 10.1093/jb/mvaa109
- ↑ Hitomi, K., Okada, R., Loo, T. M., Miyata, K., Nakamura, A. J., & Takahashi, A. (2020). DNA damage regulates senescence-associated extracellular vesicle release via the ceramide pathway to prevent excessive inflammatory responses. International Journal of Molecular Sciences, 21(10), 3720. PMID: 32466233 PMCID: PMC7279173 DOI: 10.3390/ijms21103720
- ↑ 4.0 4.1 4.2 4.3 Mas-Bargues, C., & Alique, M. (2023). Extracellular Vesicles as “Very Important Particles”(VIPs) in Aging. International Journal of Molecular Sciences, 24(4), 4250. PMID: 36835661 PMC:link DOI: link
- ↑ Yin, Y., Chen, H., Wang, Y., Zhang, L., & Wang, X. (2021). Roles of extracellular vesicles in the aging microenvironment and age‐related diseases. Journal of Extracellular Vesicles, 10(12), e12154. PMID: 34609061 PMCID: PMC8491204 DOI: 10.1002/jev2.12154
- ↑ 6.0 6.1 6.2 Lananna, B. V., & Imai, S. I. (2021). Friends and foes: Extracellular vesicles in aging and rejuvenation. FASEB bioadvances, 3(10), 787. PMID: 34632314 PMC:link DOI: link
- ↑ Jeppesen, D. K., Zhang, Q., Franklin, J. L., & Coffey, R. J. (2023). Extracellular vesicles and nanoparticles: Emerging complexities. Trends in Cell Biology. PMID: 36737375 DOI: 10.1016/j.tcb.2023.01.002
- ↑ Yu, L., Wen, H., Liu, C., Wang, C., Yu, H., Zhang, K., ... & Liu, N. (2023). Embryonic stem cell-derived extracellular vesicles rejuvenate senescent cells and antagonize aging in mice. Bioactive Materials, 29, 85-97. PMID: 37449253 PMC:10336196 DOI: 10.1016/j.bioactmat.2023.06.011
- ↑ Lehmann, B. D., Paine, M. S., Brooks, A. M., McCubrey, J. A., Renegar, R. H., Wang, R., & Terrian, D. M. (2008). Senescence-associated exosome release from human prostate cancer cells. Cancer research, 68(19), 7864-7871. PMID: 18829542 PMC:3845029 DOI: 10.1158/0008-5472.CAN-07-6538
- ↑ Alibhai, F. J., Lim, F., Yeganeh, A., DiStefano, P. V., Binesh‐Marvasti, T., Belfiore, A., ... & Li, R. K. (2020). Cellular senescence contributes to age‐dependent changes in circulating extracellular vesicle cargo and function. Aging cell, 19(3), e13103. PMID: 31960578 PMC:7059145 DOI: 10.1111/acel.13103
- ↑ Estévez‐Souto, V., Da Silva‐Álvarez, S., & Collado, M. (2022). The role of extracellular vesicles in cellular senescence. The FEBS Journal. 290(5):1203-1211 PMID: 35904466 DOI: 10.1111/febs.16585
- ↑ Zhang, Y., Kim, M. S., Jia, B., Yan, J., Zuniga-Hertz, J. P., Han, C., & Cai, D. (2017). Hypothalamic stem cells control ageing speed partly through exosomal miRNAs. Nature, 548(7665), 52-57. PMID: 28746310 PMC:5999038 DOI: 10.1038/nature23282
- ↑ Nelson, G., Wordsworth, J., Wang, C., Jurk, D., Lawless, C., Martin‐Ruiz, C., & von Zglinicki, T. (2012). A senescent cell bystander effect: senescence‐induced senescence. Aging cell, 11(2), 345-349.
- ↑ Alfonzo, M. C., Al Saedi, A., Fulzele, S., & Hamrick, M. W. (2022). Extracellular vesicles as communicators of senescence in musculoskeletal aging. JBMR plus, 6(11), e10686. PMID: 36398109 PMC:9664547 DOI: 10.1002/jbm4.10686
- ↑ Manni, G., Buratta, S., Pallotta, M. T., Chiasserini, D., Di Michele, A., Emiliani, C., ... & Fallarino, F. (2023). Extracellular Vesicles in Aging: An Emerging Hallmark?. Cells, 12(4), 527. PMID: 36831194 PMC:9954704 DOI: 10.3390/cells12040527
- ↑ Elbakrawy, E., Kaur Bains, S., Bright, S., Al-Abedi, R., Mayah, A., Goodwin, E., & Kadhim, M. (2020). Radiation-induced senescence bystander effect: The role of exosomes. Biology, 9(8), 191. PMID: 32726907 PMC:7465498 DOI: 10.3390/biology9080191
- ↑ Romero-García, N., Huete-Acevedo, J., Mas-Bargues, C., Sanz-Ros, J., Dromant, M., & Borrás, C. (2023). The Double-Edged Role of Extracellular Vesicles in the Hallmarks of Aging. Biomolecules, 13(1), 165. https://doi.org/10.3390/biom13010165
- ↑ Sun, Z., Hou, X., Zhang, J., Li, J., Wu, P., Yan, L., & Qian, H. (2022). Diagnostic and Therapeutic Roles of Extracellular Vesicles in Aging-Related Diseases. Oxidative Medicine and Cellular Longevity, 2022. PMID: 35979398 PMCID: PMC9377967 DOI: 10.1155/2022/6742792
- ↑ Sun, I. O., & Lerman, L. O. (2020). Urinary extracellular vesicles as biomarkers of kidney disease: From diagnostics to therapeutics. Diagnostics, 10(5), 311. PMID: 32429335 PMCID: PMC7277956 DOI: 10.3390/diagnostics10050311
- ↑ Yavuz, H., Weder, M. M., & Erdbrügger, U. (2023). Extracellular Vesicles in Acute Kidney Injury. Nephron, 147(1), 48-51. PMID: 36183697 DOI: 10.1159/000526842
- ↑ Erdbrügger, U., Hoorn, E. J., Le, T. H., Blijdorp, C. J., & Burger, D. (2023). Extracellular Vesicles in Kidney Diseases: Moving Forward. Kidney360, 4(2), 245-257. DOI: 10.34067/KID.0001892022
- ↑ 22.0 22.1 Sanz-Ros, J., Mas-Bargues, C., Romero-García, N., Huete-Acevedo, J., Dromant, M., & Borrás, C. (2023). Extracellular vesicles as therapeutic resources in the clinical environment. International Journal of Molecular Sciences, 24(3), 2344. PMID: 36768664 PMC:link DOI: 10.3390/ijms24032344
- ↑ Zhang, B., Tian, X., Hao, J., Xu, G., & Zhang, W. (2020). Mesenchymal stem cell-derived extracellular vesicles in tissue regeneration. Cell transplantation, 29, 0963689720908500. PMID: 32207341 PMC:link DOI: 10.1177/0963689720908500
- ↑ Tan, T. T., Toh, W. S., Lai, R. C., & Lim, S. K. (2022). Practical considerations in transforming MSC therapy for neurological diseases from cell to EV. Experimental Neurology, 349, 113953. PMID: 34921846 DOI: 10.1016/j.expneurol.2021.113953
- ↑ Tang, S., Chen, P., Zhang, H., Weng, H., Fang, Z., Chen, C., ... & Chen, X. (2021). Comparison of curative effect of human umbilical cord-derived mesenchymal stem cells and their small extracellular vesicles in treating osteoarthritis. International Journal of Nanomedicine, 16, 8185. PMID: 34938076 PMC:link DOI: 10.2147/IJN.S336062
- ↑ Zhuang, Y., Jiang, S., Yuan, C., & Lin, K. (2023). The potential therapeutic role of extracellular vesicles in osteoarthritis. Advanced biomaterials for osteochondral regeneration, 16648714, 101. PMID: 36185451 PMC:link DOI: 10.3389/fbioe.2022.1022368
- ↑ Sanz-Ros, J., Mas-Bargues, C., Romero-García, N., Huete-Acevedo, J., Dromant, M., & Borrás, C. (2022). Therapeutic Potential of Extracellular Vesicles in Aging and Age-Related Diseases. International Journal of Molecular Sciences, 23(23), 14632. PMID: 36498960 PMC:link DOI: 10.3390/ijms232314632
- ↑ Lu, Y., Huang, W., Li, M., & Zheng, A. (2023). Exosome-Based Carrier for RNA Delivery: Progress and Challenges. Pharmaceutics, 15(2), 598. PMID: 36839920 PMC:link DOI: link
- ↑ Wang, T., Zhang, J., Liao, J., Zhang, F., & Zhou, G. (2020). Donor genetic backgrounds contribute to the functional heterogeneity of stem cells and clinical outcomes. Stem Cells Translational Medicine, 9(12), 1495-1499. PMID: 32830917 PMC:link DOI: 10.1002/sctm.20-0155
- ↑ Popowski, K. D., de Juan Abad, B. L., George, A., Silkstone, D., Belcher, E., Chung, J., ... & Cheng, K. (2022). Inhalable exosomes outperform liposomes as mRNA and protein drug carriers to the lung. Extracellular Vesicle, 1, 100002. PMID: 36523538 PMC:link DOI: 10.1016/j.vesic.2022.100002
- ↑ Popowski, K. D., Moatti, A., Scull, G., Silkstone, D., Lutz, H., de Juan Abad, B. L., ... & Cheng, K. (2022). Inhalable dry powder mRNA vaccines based on extracellular vesicles. Matter, 5(9), 2960-2974. PMID: 35847197 PMC:link DOI: 10.1016/j.matt.2022.06.012
- ↑ Hofmann, B. (2018). Young blood rejuvenates old bodies: a call for reflection when moving from mice to men. Transfusion Medicine and Hemotherapy, 45(1), 67-71. PMID: 29593463 PMC:link DOI: 10.1159/000481828
- ↑ 33.0 33.1 Prattichizzo, F., Giuliani, A., Sabbatinelli, J., Mensà, E., De Nigris, V., La Sala, L., ... & Ceriello, A. (2019). Extracellular vesicles circulating in young organisms promote healthy longevity. Journal of Extracellular Vesicles, 8(1), 1656044. PMID: 31489148 PMC:link DOI: 10.1080/20013078.2019.1656044
- ↑ Dorronsoro, A., Santiago, F. E., Grassi, D., Zhang, T., Lai, R. C., McGowan, S. J., ... & Robbins, P. D. (2021). Mesenchymal stem cell‐derived extracellular vesicles reduce senescence and extend health span in mouse models of aging. Aging cell, 20(4), e13337. PMID: 33728821 PMC:link DOI: link
- ↑ Liu, S., Mahairaki, V., Bai, H., Ding, Z., Li, J., Witwer, K. W., & Cheng, L. (2019). Highly purified human extracellular vesicles produced by stem cells alleviate aging cellular phenotypes of senescent human cells. Stem Cells, 37(6), 779-790. PMID: 30811771 PMC:link DOI: link
- ↑ Lai, R. C., Arslan, F., Lee, M. M., Sze, N. S. K., Choo, A., Chen, T. S., ... & Lim, S. K. (2010). Exosome secreted by MSC reduces myocardial ischemia/reperfusion injury. Stem cell research, 4(3), 214-222. PMID: 20138817 DOI: link
- ↑ Tan, C. Y., Lai, R. C., Wong, W., Dan, Y. Y., Lim, S. K., & Ho, H. K. (2014). Mesenchymal stem cell-derived exosomes promote hepatic regeneration in drug-induced liver injury models. Stem cell research & therapy, 5, 1-14. PMID: 24915963 PMC:link DOI: 10.1186/scrt465
- ↑ Borghesan, M., Fafián-Labora, J., Eleftheriadou, O., Carpintero-Fernández, P., Paez-Ribes, M., Vizcay-Barrena, G., ... & O’Loghlen, A. (2019). Small extracellular vesicles are key regulators of non-cell autonomous intercellular communication in senescence via the interferon protein IFITM3. Cell Reports, 27(13), 3956-3971. PMID: 31242426 PMC:link DOI: 10.1016/j.celrep.2019.05.095
- ↑ Fafián-Labora, J. A., Rodríguez-Navarro, J. A., & O’Loghlen, A. (2020). Small extracellular vesicles have GST activity and ameliorate senescence-related tissue damage. Cell metabolism, 32(1), 71-86. PMID: 32574561 PMC:link DOI: 10.1016/j.cmet.2020.06.004
- ↑ Lee, B. R., Kim, J. H., Choi, E. S., Cho, J. H., & Kim, E. (2018). Effect of young exosomes injected in aged mice. International Journal of Nanomedicine, 13, 5335. PMID: 30254438 PMC:link DOI: 10.2147/IJN.S170680
- ↑ 41.0 41.1 41.2 Sanz-Ros, J., Romero-García, N., Mas-Bargues, C., Monleón, D., Gordevicius, J., Brooke, R. T., ... & Borrás, C. (2022). Small extracellular vesicles from young adipose-derived stem cells prevent frailty, improve health span, and decrease epigenetic age in old mice. Science Advances, 8(42), eabq2226. PMID 36260670 DOI: link
- ↑ Smith, E. D., Tsuchiya, M., Fox, L. A., Dang, N., Hu, D., Kerr, E. O., ... & Kennedy, B. K. (2008). Quantitative evidence for conserved longevity pathways between divergent eukaryotic species. Genome research, 18(4), 564-570. PMID: 18340043 PMC:link DOI: 10.1101/gr.074724.107
- ↑ 43.0 43.1 43.2 Fafián-Labora, J. A., Rodríguez-Navarro, J. A., & O’Loghlen, A. (2020). Small extracellular vesicles have GST activity and ameliorate senescence-related tissue damage. Cell metabolism, 32(1), 71-86. PMID: 32574561 PMC:link DOI: 10.1016/j.cmet.2020.06.004
- ↑ Zhang, X., Hou, X., Te, L., Zhongsheng, Z., Jiang, J., & Wu, X. (2022). Mesenchymal stem cells and exosomes improve cognitive function in the aging brain by promoting neurogenesis. Frontiers in Aging Neuroscience. 14:1010562 PMID: 36329874 PMC:link DOI: 10.3389/fnagi.2022.1010562
- ↑ Dabrowska, S., Andrzejewska, A., Lukomska, B., & Janowski, M. (2019). Neuroinflammation as a target for treatment of stroke using mesenchymal stem cells and extracellular vesicles. Journal of Neuroinflammation, 16(1), 178-195. PMID: 31514749 PMC:link DOI: 10.1186/s12974-019-1571-8
- ↑ Wiggins, R. C. , Glatfelter, A. , Kshirsagar, B. , & Brukman, J. (1986). Procoagulant activity in normal human urine associated with subcellular particles. Kidney International 29(2), 591–597. PMID: 3702215 DOI: 10.1038/ki.1986.39
- ↑ Dong, Y. J., Hu, J. J., Song, Y. T., Gao, Y. Y., Zheng, M. J., Zou, C. Y., ... & Xie, H. Q. (2023) Extracellular Vesicles from Urine-derived Stem Cell for Tissue Engineering and Regenerative Medicine. Tissue engineering. Part B, Reviews. PMID: 37603497 DOI: 10.1089/ten.TEB.2023.0100
- ↑ Singh, A. D., Patnam, S., Manocha, A., Bashyam, L., Rengan, A. K., & Sasidhar, M. V. (2023). Polyethylene glycol-based isolation of urinary extracellular vesicles, an easily adoptable protocol. MethodsX, 102310. PMID: 37608961 PMCID: PMC10440582 DOI: 10.1016/j.mex.2023.102310
- ↑ Konoshenko, M. Y., Lekchnov, E. A., Bryzgunova, O. E., Kiseleva, E., Pyshnaya, I. A., & Laktionov, P. P. (2021). Isolation of extracellular vesicles from biological fluids via the aggregation–precipitation approach for downstream miRNAs detection. Diagnostics, 11(3), 384. PMID: 33668297 PMC7996260 DOI: 10.3390/diagnostics11030384
- ↑ Yakubovich, E. I., Polischouk, A. G., & Evtushenko, V. I. (2022). Principles and problems of exosome isolation from biological fluids. Biochemistry (Moscow), Supplement Series A: Membrane and Cell Biology, 16(2), 115-126. PMID: 35730027 PMC9202659 DOI: 10.1134/S1990747822030096
- ↑ Erdbrügger, U., Blijdorp, C. J., Bijnsdorp, I. V., Borràs, F. E., Burger, D., Bussolati, B., ... & Martens‐Uzunova, E. S. (2021). Urinary extracellular vesicles: A position paper by the Urine Task Force of the International Society for Extracellular Vesicles. Journal of Extracellular Vesicles, 10(7). PMID: 34035881 PMC: 8138533] DOI: 10.1002/jev2.12093
- ↑ Wang, X., Hu, S., Zhu, D., Li, J., Cheng, K., & Liu, G. (2023). Comparison of extruded cell nanovesicles and exosomes in their molecular cargos and regenerative potentials. Nano Research, 1-12. https://doi.org/10.1007/s12274-023-5374-3
- ↑ Sanz-Ros, J., Mas-Bargues, C., Romero-García, N., Huete-Acevedo, J., Dromant, M., & Borrás, C. (2023). Extracellular Vesicles as Therapeutic Resources in the Clinical Environment. International Journal of Molecular Sciences, 24(3), 2344. PMID: 36768664 PMC:[https://www.ncbi.nlm.nih.gov/pmc/articles/PMC9917082 DOI: 10.3390/ijms24032344
- ↑ Fernandez-Rebollo, E., Franzen, J., Goetzke, R., Hollmann, J., Ostrowska, A., Oliverio, M., ... & Wagner, W. (2020). Senescence-associated metabolomic phenotype in primary and iPSC-derived mesenchymal stromal cells. Stem cell reports, 14(2), 201-209. PMID: 31983656 PMCID: PMC7013233 DOI: 10.1016/j.stemcr.2019.12.012
- ↑ Wang, Q., Wang, Y., Chang, C., Ma, F., Peng, D., Yang, S., ... & Zhou, G. (2023). Comparative analysis of mesenchymal stem/stromal cells derived from human induced pluripotent stem cells and the cognate umbilical cord mesenchymal stem/stromal cells. Heliyon, 9(1):e12683. PMID: 36647346 PMCID: PMC9840238 DOI: 10.1016/j.heliyon.2022.e12683
- ↑ Levy D., Abadchi S., Shababi N., ... & Jay S.M. (2023). Induced pluripotent stem cell-derived extracellular vesicles promote wound repair in a diabetic mouse model via an anti-inflammatory immunomodulatory mechanism. bioRxiv https://doi.org/10.1101/2023.03.19.533334
- ↑ Sun, H., Wang, X., Pratt, R. E., Hodgkinson, C., & Dzau, V. (2023). C166 Exosomes are a Novel Fibroblast Selective Delivery System Which Potentiates miR Cardiac Reprogramming. JACC: Basic to Translational Science. Available at SSRN 4279247. http://dx.doi.org/10.2139/ssrn.4279247
- ↑ Sun, H., Pratt, R. E., Dzau, V. J., & Hodgkinson, C. P. (2023). Neonatal and adult cardiac fibroblasts exhibit inherent differences in cardiac regenerative capacity. Journal of Biological Chemistry, 104694. PMID: 37044217 DOI: 10.1016/j.jbc.2023.104694
- ↑ Danilushkina, A. A., Emene, C. C., Barlev, N. A., & Gomzikova, M. O. (2023). Strategies for Engineering of Extracellular Vesicles. International Journal of Molecular Sciences, 24(17), 13247. https://doi.org/10.3390/ijms241713247
- ↑ Hertel, F. C., Silva, A. S. D., Sabino, A. D. P., Valente, F. L., & Reis, E. C. C. (2022). Preconditioning methods to improve mesenchymal stromal cell-derived extracellular vesicles in bone regeneration—a systematic review. Biology, 11(5), 733. PMID: 35625461 PMC9138769 DOI: 10.3390/biology11050733
- ↑ Haupt, M., Gerner, S. T., Huttner, H. B., & Doeppner, T. R. (2023). Preconditioning Concepts for the Therapeutic Use of Extracellular Vesicles Against Stroke. Stem Cells Translational Medicine, 12(11), 707-713. PMID: 37696005 DOI: 10.1093/stcltm/szad055
- ↑ Ala, M. (2023). The beneficial effects of mesenchymal stem cells and their exosomes on myocardial infarction and critical considerations for enhancing their efficacy. Ageing Research Reviews, 101980. PMID: 37302757 DOI: 10.1016/j.arr.2023.101980
- ↑ Ning, Y., Huang, P., Chen, G., Xiong, Y., Gong, Z., Wu, C., ... & Yang, Y. (2023). Atorvastatin-pretreated mesenchymal stem cell-derived extracellular vesicles promote cardiac repair after myocardial infarction via shifting macrophage polarization by targeting microRNA-139-3p/Stat1 pathway. BMC medicine, 21(1), 1-18. PMID: 36927608 PMC10022054 DOI: 10.1186/s12916-023-02778-x
- ↑ Liu, W., Li, L., Rong, Y., Qian, D., Chen, J., Zhou, Z., Luo, Y., Jiang, D., Cheng, L., Zhao, S., et al. (2020). Hypoxic mesenchymal stem cell-derived exosomes promote bone fracture healing by the transfer of miR-126. Acta Biomater. 103, 196–212. https://doi.org/10.1016/j.actbio.2019.12.020.
- ↑ Chang, L. H., Wu, S. C., Chen, C. H., Chen, J. W., Huang, W. C., Wu, C. W., ... & Ho, M. L. (2023). Exosomes Derived from Hypoxia-Cultured Human Adipose Stem Cells Alleviate Articular Chondrocyte Inflammaging and Post-Traumatic Osteoarthritis Progression. International Journal of Molecular Sciences, 24(17), 13414. PMID: 37686220 PMC10487932 DOI: 10.3390/ijms241713414
- ↑ Deng, J., Wang, X., Zhang, W., Sun, L., Han, X., Tong, X., ... & Liu, Y. (2023). Versatile hypoxic extracellular vesicles laden in an injectable and bioactive hydrogel for accelerated bone regeneration. Advanced Functional Materials, 33(21), 2211664. https://doi.org/10.1002/adfm.202211664
- ↑ Xu, D., & Tahara, H. (2013). The role of exosomes and microRNAs in senescence and aging. Advanced drug delivery reviews, 65(3), 368-375. PMID: 22820533 DOI: link
- ↑ Takasugi, M. (2018). Emerging roles of extracellular vesicles in cellular senescence and aging. Aging cell, 17(2), e12734. DOI: link
- ↑ Urbanelli, L., Buratta, S., Sagini, K., Tancini, B., & Emiliani, C. (2016). Extracellular vesicles as new players in cellular senescence. International Journal of Molecular Sciences, 17(9), 1408. PMID: 27571072 PMC:link DOI: link
- ↑ Oh, C., Koh, D., Jeon, H. B., & Kim, K. M. (2022). The Role of Extracellular Vesicles in Senescence. Molecules and Cells, 45(9), 603-609. PMID: 36058888 PMC:link DOI: 10.14348/molcells.2022.0056
- ↑ Kostyushev, D., Kostyusheva, A., Brezgin, S., Smirnov, V., Volchkova, E., Lukashev, A., & Chulanov, V. (2020). Gene Editing by Extracellular Vesicles. International Journal of Molecular Sciences, 21(19), 7362. PMID 33028045 DOI: link
- ↑ D’Anca, M., Fenoglio, C., Serpente, M., Arosio, B., Cesari, M., Scarpini, E. A., & Galimberti, D. (2019). Exosome determinants of physiological aging and age-related neurodegenerative diseases. Frontiers in aging neuroscience, 11, 232. PMID: 31555123 PMC:link DOI: link
- ↑ Guix, F. X. (2020). The interplay between aging‐associated loss of protein homeostasis and extracellular vesicles in neurodegeneration. Journal of neuroscience research, 98(2), 262-283. PMID: 31549445 DOI: link
- ↑ Misawa, T., Tanaka, Y., Okada, R., & Takahashi, A. (2020). Biology of extracellular vesicles secreted from senescent cells as senescence‐associated secretory phenotype factors. Geriatrics & Gerontology International, 20(6), 539-546. PMID: 32358923 DOI: link

This article is currently a draft.
Material may not yet be complete, information may presently be omitted, and certain parts of the content may be subject to radical, rapid alteration. More information pertaining to this may be available on the talk page.