Longevity of insects with social castes
Social insects such as ants,[1] bees,[2] and termites,[3] have a distinct division of labor among individuals within their colonies. The colony is typically composed of a reproductive queen or queens and non-reproductive workers or soldier castes.[4] Research has shown that there are significant differences in longevity between the queen and worker castes in many social insect species. In general, queens live much longer than workers, and any worker can become a queen given the appropriate environmental cues by turning on or off specific genes (i.e. via epigenetic mechanisms).[5]
The difference in longevity of the social castes is thought to be related to the different roles that queens and workers play within the colony. Queens are responsible for reproducing and maintaining the colony, so they have evolved to live longer in order to increase their chances of reproducing.[6] In contrast, workers are responsible for foraging food and caring for the colony's young, so they have evolved to have shorter lifespans as they expend a lot of energy and are more prone to injuries and predation.[6] Additionally, queen ants and bees have a different hormonal regulation that allows them to live longer, and different gene expression profiles that increases their resistance to stress, while reducing their metabolism.
The fact that queens live many times longer than workers, despite sharing the same genome, provides a particularly useful model for understanding the epigenetic pathways and mechanisms that influence aging.[5]
Longevity of workers and queens
Bees
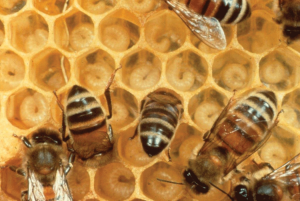
In hives, queen bees are normally the only female who reproduces, whereas the vast majority of bees are sterile females known as worker bees. Queen honeybees can live for several years, while workers typically only live for several months.[6]
Even in a normal hive, about 1% of workers have ovaries developed enough to lay eggs. A laying worker bee is a worker bee that lays unfertilized eggs, usually in the queenless colonies. In colonies of the neotropical stingless bee Scaptotrigona aff. postica, the lifespan of worker bees was about a third longer in hives without mated queens (known as non-queenright colonies).[8]
The worker bees of this species substantially alter their behavior without mated queens, for example by spending more time inside the nest building brood cells rather than participating in more risky foraging behaviors, therefore causing an increase in their overall longevity. Workers also start to lay haploid eggs, allowing some direct reproduction by workers in the absence of a queen.[8] This phenomenons highlight the plasticity of longevity in honeybees depending on environmental stimuli.
Ants
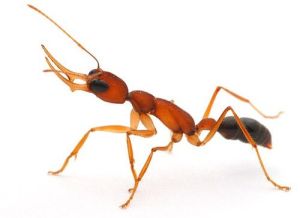
Ants also display a remarkable plasticity in their longevity that can be regulated via epigenetic mechanisms.[5] For instance, in the ant species L. niger, queen ants live for 20 to 30 years, workers for 1 to 3 years and males for only a few weeks.[9]
Gamergate ants
While in most ant species social castes are permanently established during the larval stage, adult Harpegnathos saltator workers can acquire a queen-like phenotype and become reproductive individuals called “gamergates”.[10] Workers that become gamergates also attain queen-like longevity, with a fivefold increase in average life span from 7 months to 3 years.[11]
In the ant Harpegnathos saltator, aging workers exhibited a progressive loss of ensheathing glia cells, whereas in gamergates, they remained a larger proportion of total glia cells over the same time span. Consistent with this, old gamergates retained the ability to mount a response to neuronal damage, which was lost in old workers.[12]
It was found that gamergates have elevated expression of gamergate-specific gene encoding a truncated form of a heat shock factor (HSF) most similar to mammalian HSF2 (hsalHSF2). In workers, hsalHSF2 was bound to DNA only upon heat stress, whereas in gamergates, hsalHSF2 bound to DNA even in the absence of heat stress and was localized to gamergate-biased heat shock response (HSR) genes. Expression of hsalHSF2 in Drosophila melanogaster led to enhanced heat shock survival and extended life span in the absence of heat stress.[13]
Tapeworm-infected ants
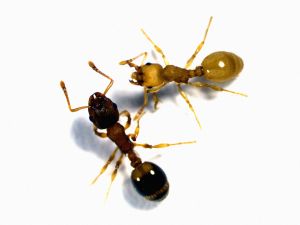
Parasite infection induces multiple alterations in the adult workers. After infection, the survival of infected workers is increased compared to their uninfected nest-mates.[15] [16] A parasitic tapeworm greatly lengthens the lives of its ant hosts by secreting a rich cocktail (more than 250 proteins) of antioxidants and other compounds.
Most secreted proteins from these parasitic tapeworms don't have any known annotated orthologs, which is indicative of potential novel functions and a long history of adaptation within this species interaction.[17]
A study found that both queens and infected workers express more of a gene called silver (carboxypeptidase D), compared to uninfected workers.[16] Researchers previously linked the silver gene to an extended lifespan in fruit flies.[18][19] However, a possible bias could be that infected workers do not engage in foraging outside the nest, and therefore have lower extrinsic mortality similar to queens, which also stay inside the nest.[16]
Another study[20] found two proteins exclusively in infected workers and healthy queens: fatty acid-binding protein 1 and facilitated trehalose transporter Tret1-like - the protein that is responsible for transporting trehalose, the main source of energy for insects, from the fat body into the haemolymph. One of trehalose's functions is the protection from different environmental stressors, such as oxidative stress. In addition, four unique proteins (death-associated protein 1 (which positively regulates apoptosis); chymotrypsin-2 (shown to extend lifespan in Drosophila) and two uncharacterized proteins) were found in infected workers.
Wolbachia-infected ants
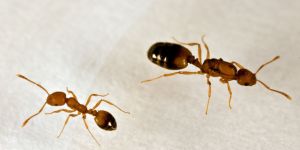
Wolbachia, a widespread maternally-inherited endosymbiotic bacteria, infects an estimated one-third of all ant species. In infected Monomorium pharaonis colonies, queens produce more eggs. Despite increased egg-laying by queens and higher colony-level metabolic rates, Wolbachia infection was not associated with decreased queen lifespan. In fact, in workers, which are obligately sterile, Wolbachia infection was associated with longer lifespan.[22] Thus, increased egg-laying rates by queens and longer worker lifespans contribute to the higher growth rate and productivity that characterizes Wolbachia-infected colonies.
A role of metabolic provisioning of the host by endosymbionts has been demonstrated in several empirical studies. For example, Wolbachia can increase female fecundity in Drosophila flies by influencing iron homeostasis. In filarial nematodes, Wolbachia provisions the host with heme and riboflavin. Likewise, in a spider species, synthesis of fat and free amino acids has been shown to be improved by a Wolbachia infection, and in bedbugs, Wolbachia plays a nutritional role in vitamin B synthesis.[23][24]
Termites
Termites (Isoptera) are pale-coloured, soft-bodied eusocial insects living in complex societies that are characterized by a caste system in which despite the fact that all members in a termite colony have the same genetic background, few reproductively mature individuals (including a queen and a sperm-producing kings) reproduce and have extraordinarily long lives, while the large majority (non-reproductives, including workers and soldiers) perform tasks such as foraging, brooding and defence.[25][26] Unlike hymenopteran social insects (ants, bees and wasps), where the sterile workers are all female, in termites both males and females can be workers.[27] Furthermore, workers have unique flexibility in that a worker has the capability to develop into apterous neotenic reproductives that develop in the absence of reproductives to provide for continued growth of the colony.[28]
Although the question of how termite queens, which exhibit (as well as ants and honeybees) extraordinary longevity and fertility in comparison with non-reproductive individuals has attracted much attention, the molecular mechanisms involved are not yet understood. [30] It has been hypothesized that the queens of the termite Reticulitermes speratus have high level of oxidative stress resistance and suffer lower levels of oxidative damage than non-reproductive workers, due to the a highly efficient antioxidant system. In particular, queens had two times higher catalase activity and more than seven times higher expression levels of the catalase gene RsCAT1 than workers. Queens also showed higher expression levels of the peroxiredoxin gene RsPRX6.[31]
An important antioxidant contributing to survival in termites is uric acid, which is reserved and used as a valuable nitrogen source. Uric acid was shown to be provided by workers to reproductive castes.[32][33] It was found that king- and queen-specific degradation of uric acid contributes to reproduction and longevity in the subterranean termite Reticulitermes speratus. The urate oxidase gene (RsUAOX), which catalyses the first step of nitrogen recycling from stored uric acid, was highly expressed in mature kings and queens, and upregulated with differentiation into neotenic kings/queens.[34]
The haploid genome sizes (C-values) of termites (Isoptera) ranged from 0.58 to 1.90 pg, (1pg = 978 Mb).[37]
Mechanisms of exceptional longevity
Some of the most prominent mechanisms thought to underly the extended longevity of queens are:
Endocrine signalling and insulin binding protein Imp-L2
Imaginal morphogenesis protein‐Late 2 (Imp-L2), a putative homolog of vertebrate insulin-like growth factor (IGF)-binding protein 7 (IGFBP7), counteracts insulin/IGF signalling (IIS) in Drosophila and is essential for starvation resistance.[39] It is known that genetic manipulations of pathway components that result in dampened IIS extend lifespan in worms, flies and mice, and ameliorate age-dependent functional decline in a FOXO-dependent manner.[40] Increased IMP-L2 levels in Drosophila similarly results in substantial lifespan extension.[41]
It is assumed that in the process of egg-laying, some ant queens produce Imp-L2, which suppresses the aging effects of insulin, so that they can consume all the additional food needed for their egg-laying without shortening their lives.[42] As is known, reducing the AKT (also known as Protein kinase B) branch of insulin signaling in the fat body and adipose tissue lengthens the life of Drosophila.[43] A study showed that strong AKT phosphorylation after insulin treatment was completely suppressed by Imp-L2.[42]
Oxidative stress resistance
Vitellogenin (Vtg or Vg) is an egg yolk precursor produced by nearly all egg-laying females species. It is a source of nutrient and has additional roles in the scavenging of reactive oxygen species (ROS).[44] The expression of vitellogenin (Vg) appears to be linked to the queens increased resistance to stress.[45] Bees with higher Vg levels had increased survival, while reducing the expression of Vg led to decreased lifespan. However, in queen honey bees, the expression of antioxidant genes is not necessarily increased nor is required for their long lifespan.[46]
Genes underlying the reproductive division of labor
For identifying differences in gene expression between queens and workers in eusocial insects pairs of the queen and worker RNA sequencing data were used to calculate the queen/worker ratio referred to as the QW ratio.[47] Meta-analysis of RNA-seq data revealed 20 genes with differential expression between queens and workers. Among these genes, vitellogenin and vitellogenin receptors, which are highly expressed in queens across many social insects. SPARC (secreted protein acidic and cysteine rich) was highly expressed in queens, and RSG7 (regulator of G protein signaling 7) was highly expressed in workers. In mice, SPARC promotes insulin secretion via down-regulation of regulator of G protein 4 (RGS4) expression in pancreatic β cells. Functional analyses of the 20 genes retrieved from massive datasets of a large number of eusocial species may reveal in the future key regulators of the reproductive division of labor and and multiple differences in life expectancy.
When workers leave the natal colony or a queen dies, the expression of stage-specific genes is induced in workers, which leads to the differentiation of workers to reproductives.[48] In particular the relative expression level of Ras in the isolated female workers (IWs) was 131-fold than that of the workers, which indicated that Ras was especially overexpressed in workers in the absence of queens for activation of the Ras-ERK signalling pathway to drive the ovary development of isolated workers.[48] It was suggested that the signal transduction along the Ras-MAPK pathway crucially controls the reproductive plasticity of the workers and short-lived individuals can become long-lived individuals by the transition of castes.[48]
Effective transposon regulation
For termites but not for hymenopterans, it was found that caste and age was associated with the expression of transposable elements (TEs). While sterile workers who live only weeks had substantially higher TE activity, TE expression did not increase with age in the fat body of queens, despite a sevenfold increase in overall gene expression, due to a significant upregulation of the piRNA-pathway in 20-year-old queens of Macrotermes natalensis.[49][50]
References
- ↑ Majoe, M., Libbrecht, R., Foitzik, S., & Nehring, V. (2021). Queen loss increases worker survival in leaf-cutting ants under paraquat-induced oxidative stress. Philosophical Transactions of the Royal Society B, 376(1823), 20190735. PMID: 33678018 PMC link DOI: 10.1098/rstb.2019.0735
- ↑ Shell, W. A., & Rehan, S. M. (2022). Social divergence: molecular pathways underlying castes and longevity in a facultatively eusocial small carpenter bee. Proceedings of the Royal Society B, 289(1971), 20212663. PMID: 35317677 PMC link DOI: 10.1098/rspb.2021.2663
- ↑ Li, Y. X., Ye, C. X., Su, J., Nabi, G., Su, X. H., & Xing, L. X. (2022). De Novo Transcriptome Assembly and Analysis of Longevity Genes Using Subterranean Termite (Reticulitermes chinensis) Castes. International Journal of Molecular Sciences, 23(21), 13660. PMID: 36362447 PMC link DOI: 10.3390/ijms232113660
- ↑ Opachaloemphan, C., Yan, H., Leibholz, A., Desplan, C., & Reinberg, D. (2018). Recent advances in behavioral (epi) genetics in eusocial insects. Annual review of genetics, 52, 489. PMID: 30208294 PMC link DOI: 10.1146/annurev-genet-120116-024456
- ↑ 5.0 5.1 5.2 Sieber, K. R., Dorman, T., Newell, N., & Yan, H. (2021). (Epi) genetic mechanisms underlying the evolutionary success of eusocial insects. Insects, 12(6), 498. PMID: 34071806 PMC link DOI: 10.3390/insects12060498
- ↑ 6.0 6.1 6.2 Remolina, S. C., & Hughes, K. A. (2008). Evolution and mechanisms of long life and high fertility in queen honey bees. Age, 30(2), 177-185.
- ↑ https://www.beeculture.com/laying-workers-happens-fix/
- ↑ 8.0 8.1 dos Santos Conceição Lopes, B., Campbell, A. J., & Contrera, F. A. L. (2020). Queen loss changes behavior and increases longevity in a stingless bee. Behavioral Ecology and Sociobiology, 74, 1-9. DOI: 10.1007/s00265-020-2811-8
- ↑ Jemielity, S., Chapuisat, M., Parker, J. D., & Keller, L. (2005). Long live the queen: studying aging in social insects. Age, 27(3), 241-248. PMID: 23598656 PMC link DOI: 10.1007/s11357-005-2916-z
- ↑ Peeters, C., Liebig, J., & Hölldobler, B. (2000). Sexual reproduction by both queens and workers in the ponerine ant Harpegnathos saltator. Insectes Sociaux, 47, 325-332. https://doi.org/10.1007/PL00001724
- ↑ Ghaninia, M., Haight, K., Berger, S. L., Reinberg, D., Zwiebel, L. J., Ray, A., & Liebig, J. (2017). Chemosensory sensitivity reflects reproductive status in the ant Harpegnathos saltator. Scientific reports, 7(1), 3732. PMID: 28623371 PMC link DOI: 10.1038/s41598-017-03964-7
- ↑ Sheng, L., Shields, E. J., Gospocic, J., Glastad, K. M., Ratchasanmuang, P., Berger, S. L., ... & Bonasio, R. (2020). Social reprogramming in ants induces longevity-associated glia remodeling. Science advances, 6(34), eaba9869. PMID: 32875108 PMC link DOI: 10.1126/sciadv.aba9869
- ↑ Glastad, K. M., Roessler, J., Gospocic, J., Bonasio, R., & Berger, S. L. Long ant life span is maintained by a unique heat shock factor. Genes & development. 37(9-10), 398-417 doi: 10.1101/gad.350250.122
- ↑ https://www.beeculture.com/laying-workers-happens-fix/
- ↑ Beros, S., Lenhart, A., Scharf, I., Negroni, M. A., Menzel, F., & Foitzik, S. (2021). Extreme lifespan extension in tapeworm-infected ant workers. Royal Society open science, 8(5), 202118. PMID: 34017599 PMC link DOI: 10.1098/rsos.202118
- ↑ 16.0 16.1 16.2 Stoldt, M., Klein, L., Beros, S., Butter, F., Jongepier, E., Feldmeyer, B., & Foitzik, S. (2021). Parasite presence induces gene expression changes in an ant host related to immunity and longevity. Genes, 12(1), 95. PMID: 33451085 PMC link DOI: 10.3390/genes12010095
- ↑ Hartke, J., Ceron-Noriega, A., Stoldt, M., Sistermans, T., Kever, M., Fuchs, J., ... & Foitzik, S. (2022). What doesn't kill you makes you live longer-Longevity of a social host linked to parasite proteins. bioRxiv. doi: 10.1101/2022.12.23.521666
- ↑ Pauls, D., Hamarat, Y., Trufasu, L., Schendzielorz, T. M., Gramlich, G., Kahnt, J., ... & Wegener, C. (2019). Drosophila carboxypeptidase D (SILVER) is a key enzyme in neuropeptide processing required to maintain locomotor activity levels and survival rate. European Journal of Neuroscience, 50(9), 3502-3519. PMID: 31309630 DOI: 10.1111/ejn.14516
- ↑ Carnes, M. U., Campbell, T., Huang, W., Butler, D. G., Carbone, M. A., Duncan, L. H., ... & Mackay, T. F. (2015). The genomic basis of postponed senescence in Drosophila melanogaster. PLoS One, 10(9), e0138569. PMID: 26378456 PMC link DOI: 10.1371/journal.pone.0138569
- ↑ Hartke, J., Ceron‐Noriega, A., Stoldt, M., Sistermans, T., Kever, M., Fuchs, J., ... & Foitzik, S. (2023). Long live the host! Proteomic analysis reveals possible strategies for parasitic manipulation of its social host. Molecular ecology, 32(21), 5877-5889. https://doi.org/10.1111/mec.17155
- ↑ https://www.beeculture.com/laying-workers-happens-fix/
- ↑ Rohini Singh, Sachin Suresh, Jennifer Fewell, Jon Harrison and Timothy Linksvayer (2023). Wolbachia-infected pharaoh ant colonies have higher egg production, metabolic rate, and worker survival. bioRxiv doi: 10.1101/2023.01.31.526493
- ↑ Katlav, A., Nguyen, D. T., Morrow, J. L., Spooner-Hart, R. N., & Riegler, M. (2022). Endosymbionts moderate constrained sex allocation in a haplodiploid thrips species in a temperature-sensitive way. Heredity, 128(3), 169-177. PMID: 35115648 PMC link DOI: 10.1038/s41437-022-00505-5
- ↑ Katlav, A., Cook, J. M., & Riegler, M. (2022). Common endosymbionts affect host fitness and sex allocation via egg size provisioning. Proceedings of the Royal Society B, 289(1971), 20212582. PMID: 35350856 PMC link DOI: 10.1098/rspb.2021.2582
- ↑ Korb, J. (2007). Termites. Current Biology, 17(23), R995-R999. PMID: 18054770 DOI: 10.1016/j.cub.2007.10.033
- ↑ Korb, J., Poulsen, M., Hu, H., Li, C., Boomsma, J. J., Zhang, G., & Liebig, J. (2015). A genomic comparison of two termites with different social complexity. Frontiers in Genetics, 6, 9. PMID: 25788900 PMC link DOI: 10.3389/fgene.2015.00009
- ↑ Revely, L., Sumner, S., & Eggleton, P. (2021). The plasticity and developmental potential of termites. Frontiers in Ecology and Evolution, 9, 552624. https://doi.org/10.3389/fevo.2021.552624
- ↑ Miura, T., Scharf, M. E., Bignell, D. E., Roisin, Y., & Lo, N. (2011). Biology of termites: A modern synthesis. Molecular Basis Underlying Caste Differentiation in Termites pp 211–253 Roisin, Nathan Lo DOI:10.1007/978-90-481-3977-4_9
- ↑ Harrison, M. C., Jongepier, E., Robertson, H. M., Arning, N., Bitard-Feildel, T., Chao, H., ... & Bornberg-Bauer, E. (2018). Hemimetabolous genomes reveal molecular basis of termite eusociality. Nature ecology & evolution, 2(3), 557-566. PMID: 29403074 PMC link DOI: 10.1038/s41559-017-0459-1
- ↑ Tasaki, E., Takata, M., & Matsuura, K. (2021). Why and how do termite kings and queens live so long?. Philosophical Transactions of the Royal Society B, 376(1823), 20190740. PMID: 33678028 PMC link DOI: 10.1098/rstb.2019.0740
- ↑ Tasaki, E., Kobayashi, K., Matsuura, K., & Iuchi, Y. (2017). An efficient antioxidant system in a long-lived termite queen. PLoS One, 12(1), e0167412. PMID: 28076409 PMC link DOI: 10.1371/journal.pone.0167412
- ↑ Tong, R. L., Patel, J. S., Gordon, J. M., Lee, S. B., Chouvenc, T., & Su, N. Y. (2023). Exuviae Recycling Can Enhance Queen Oviposition and Colony Growth in Subterranean Termites (Blattodea: Rhinotermitidae: Coptotermes). Environmental Entomology, nvad009. PMID: 36773009 DOI: 10.1093/ee/nvad009
- ↑ Tasaki, E., Sakurai, H., Nitao, M., Matsuura, K., & Iuchi, Y. (2017). Uric acid, an important antioxidant contributing to survival in termites. PLoS One, 12(6), e0179426. PMID: 28609463 PMC link DOI: 10.1371/journal.pone.0179426
- ↑ Konishi, T., Tasaki, E., Takata, M., & Matsuura, K. (2023). King-and queen-specific degradation of uric acid contributes to reproduction in termites. Proceedings of the Royal Society B, 290(1990), 20221942. PMID: 36598016 PMC link (available on 2024-01-11) DOI: 10.1098/rspb.2022.1942
- ↑ Ma, X. M., Li, Y. X., Zhang, H. X., Liu, Q., Su, X. H., & Xing, L. X. (2020). Transcriptomic evidence that insulin signalling pathway regulates the ageing of subterranean termite castes. Scientific Reports, 10(1), 1-13. PMID: 32424344 PMC link DOI: 10.1038/s41598-020-64890-9
- ↑ Séité, S., Harrison, M. C., Sillam-Dussès, D., Lupoli, R., Van Dooren, T. J., Robert, A., ... & Vasseur-Cognet, M. (2022). Lifespan prolonging mechanisms and insulin upregulation without fat accumulation in long-lived reproductives of a higher termite. Communications Biology, 5(1), 44. PMID: 35027667 PMC link DOI: 10.1038/s42003-021-02974-6
- ↑ Monroy Kuhn, J. M., Meusemann, K., & Korb, J. (2019). Long live the queen, the king and the commoner? Transcript expression differences between old and young in the termite Cryptotermes secundus. PLoS One, 14(2), e0210371. PMID: 30759161 PMC link DOI: 10.1371/journal.pone.0210371
- ↑ Harrison, M. C., Dohmen, E., George, S., Sillam-Dussès, D., Séité, S., & Vasseur-Cognet, M. (2022). Complex regulatory role of DNA methylation in caste-and age-specific expression of a termite. Open Biology, 12(7), 220047.PMID: 35857972 PMC link DOI: 10.1098/rsob.220047
- ↑ Honegger, B., Galic, M., Köhler, K., Wittwer, F., Brogiolo, W., Hafen, E., & Stocker, H. (2008). Imp-L2, a putative homolog of vertebrate IGF-binding protein 7, counteracts insulin signaling in Drosophila and is essential for starvation resistance. Journal of biology, 7, 1-11. PMID: 18412985 PMC link DOI: 10.1186/jbiol72
- ↑ Piper, M. D. W., Selman, C., McElwee, J. J., & Partridge, L. (2008). Separating cause from effect: how does insulin/IGF signalling control lifespan in worms, flies and mice?. Journal of internal medicine, 263(2), 179-191. PMID: 18226095 DOI: 10.1111/j.1365-2796.2007.01906.x
- ↑ Alic, N., Hoddinott, M. P., Vinti, G., & Partridge, L. (2011). Lifespan extension by increased expression of the Drosophila homologue of the IGFBP7 tumour suppressor. Aging cell, 10(1), 137-147. PMID: 21108726 PMC link DOI: 10.1111/j.1474-9726.2010.00653.x
- ↑ 42.0 42.1 Yan, H., Opachaloemphan, C., Carmona-Aldana, F., Mancini, G., Mlejnek, J., Descostes, N., ... & Reinberg, D. (2022). Insulin signaling in the long-lived reproductive caste of ants. Science, 377(6610), 1092-1099. PMID: 36048960 PMC link DOI: 10.1126/science.abm8767
- ↑ Cheng, X., Xie, M., Luo, L., Tian, Y., Yu, G., Wu, Q., ... & Yang, M. (2022). Inhibitor GSK690693 extends Drosophila lifespan via reduce AKT signaling pathway. Mechanisms of Ageing and Development, 202, 111633. PMID: 35065134 DOI: 10.1016/j.mad.2022.111633
- ↑ Havukainen H, Münch D, Baumann A, Zhong S, Halskau Ø, Krogsgaard M, Amdam GV. Vitellogenin recognizes cell damage through membrane binding and shields living cells from reactive oxygen species. J Biol Chem. 2013 Sep 27;288(39):28369-81. doi: 10.1074/jbc.M113.465021.
- ↑ Seehuus SC, Norberg K, Gimsa U, Krekling T, Amdam GV (2006) Reproductive protein protects functionally sterile honey bee workers from oxidative stress. Proc Natl Acad Sci USA 103:962–967
- ↑ Corona M, Hughes KA, Weaver DB, Robinson GE (2005) Gene expression patterns associated with queen honey bee longevity. Mech Ageing Dev 126:1230–1238
- ↑ Toga, K., & Bono, H. (2022). Meta-analysis of public RNA sequencing data of queens and workers in social Hymenoptera and termites. bioRxiv, 2022-11. Doi:10.1101/2022.11.20.516280
- ↑ 48.0 48.1 48.2 Ye, C., Rasheed, H., Ran, Y., Yang, X., Xing, L., & Su, X. (2019). Transcriptome changes reveal the genetic mechanisms of the reproductive plasticity of workers in lower termites. BMC Genomics, 20.702. PMID: 31500567 PMC link DOI: 10.1186/s12864-019-6037-y
- ↑ Post, F., Bornberg‐Bauer, E., Vasseur‐Cognet, M., & Harrison, M. C. (2023). More effective transposon regulation in fertile, long‐lived termite queens than in sterile workers. Molecular Ecology, 32(2), 369-380. PMID: 36320186 DOI: 10.1111/mec.16753
- ↑ Elsner, D., Meusemann, K., & Korb, J. (2018). Longevity and transposon defense, the case of termite reproductives. Proceedings of the National Academy of Sciences, 115(21), 5504-5509. PMID: 29735660 PMC link DOI: 10.1073/pnas.1804046115