Protein restriction
Reduced intake of energy (calorie restriction) has a long history in longevity research, but for almost as long scientists have been interested in understanding if restriction of specific types of macronutrients would recapitulate the effects of CR. The results of many early studies were mixed, likely due to differences in dietary protein quality and the degree of restriction.[1] However, since the end of the last century, interest in protein restriction (PR) as an intervention has been rekindled by studies which have shown that in flies and mice, total protein restriction or restriction of specific essential amino acids can extend lifespan independently of calorie intake.[2][3]
Biological mechanism
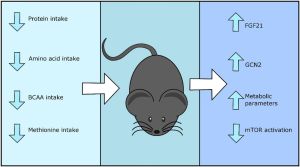
Dietary conditions of protein restriction lead to a significant increase in circulating levels of fibroblast growth factor 21 (FGF21), an insulin-sensitizing hormone. Studies in mice and rats show that circulating FGF21 is increased 10-fold within 24 hours of PR and causes subsequent activation of eukaryotic initiation factor 2α (eIF2α) in the liver through the general control nonderepresssible 2 (GNC2) kinase.[5] FGF21 appears responsible for the metabolic remodelling associated to PR, namely reduced body weight, energy expenditure and altered food intake, as these outcomes are not observed in FGF21-deficient animals subjected to PR.[5] The transcription factor ATF4 is required to upregulate FGF21 and other genes necessary to respond to PR and amino acid restriction.[6]
Interestingly, increases in the circulating levels of FGF21 are not observed in PR diets with reduced BCAAs, suggesting this form of PR might function via a metabolically distinct pathway.[7] Branched-chain amino acids or BCAAs (leucine, isoleucine and valine) are among the nine essential amino acids for humans and have an aliphatic side-chain with a branch. BCAA dietary restriction extends lifespan in fruit flies and mice by modulating the mTOR signalling pathway, the target of rapamycin.[8] Sestrin, an inhibitor of mTOR complex 1 (mTORC1), has recently been implicated as a sensor of amino acids in the intestine, and has been shown to maintain homeostasis and regulate lifespan in flies.[9]
Restricting methionine, another essential amino acid in humans, by approximately 70-80% has also been shown to be sufficient to increase lifespan in mice and to provide some of the health benefits associated to caloric restriction (CR).[10][11] Methionine restriction is hypothesized to be mediated via FGF21 and to inhibit mTORC1, although this might occur in a sexually dimorphic way and be exclusive to male mice.[12][13][14]
The relationship between amino acid intake and metabolic health is complex and no clear consensus exists yet as to the exact mechanism through which benefit might be derived, as well as its effect in other less studied tissues such as the brain.
Human studies
A number of long-term studies suggest that lower protein diets in humans are associated to improved metabolic health and increased lifespan.
NHANES III
A restrospective cohort study based in the National Health And Nutrition Examination Survey (NHANES) III, surveyed 6,381 men and women located in the USA and of 50 years old and over. They found that participants of 50 to 65 years old with high animal-derived protein intake had a 75% increased risk in mortality and a 4-fold increase in risk of age-associated diseases such as cancer.[15] However, the reverse trend was observed in participants aged over 65 years old, in which high animal-derived protein intake was associated to lower cancer risk and mortality. Independently of age, high protein intake was associated to a 5-fold increase in the risk of diabetes, although it is important to observe that the particular sample size for diabetes-related deaths was low (n=21). Of note, this study controlled for age, sex, waist circumference and total calories consumption, but did not control for amount of exercise.
Interestingly, the relationships between protein intake, health and mortality risk were no longer observed if protein consumed was plant-derived. When controlling for plant-derived protein, no positive nor negative associations were observed, suggesting that plant-derived protein has no added health benefits but rather that animal-derived protein has deleterious health effects. These findings are in agreement with previous studies showing consumption of red meat is associated to higher risk of all-cause mortality and cancer.[16]
This study also measured levels of insulin-like growth factor 1 (IGF-1) in a subset of the cohort (n= 2,253 subjects) and found that low protein intake was associated with a significant reduction in IGF-1 and in turn with a lower incidence of cancer. Higher circulating levels of IGF-1, the molecule that negatively regulates the longevity gene FOXO, has been extensively associated with an increased risk of cancer and might act as a promoter of tumorigenesis.[17][18][19]
(EPIC)-NL
A large prospective cohort study based on the European Prospective Investigation into Cancer and Nutrition (EPIC)-NL study, measured dietary protein intake and incidence of diabetes among 38,094 participants in Europe.[20] Similarly to the NHANES III study, they found that higher animal protein intake was associated with increased risk of diabetes. However, in discrepancy to the NHANES III study, the (EPIC)-NL also found an association, although more nuanced, between higher total protein intake and increased incidence of diabetes which was independent of protein source.
RCTs
A small randomised controlled trial (RCT) showed that moderate protein restriction (PR) improved several health markers in both humans and mice.[7] They observed that 7-9% PR led to improved blood glucose levels and blood glucose tolerance, while decreasing body mass index (BMI) and fat mass in both humans and mice. However, the human subjects of the study (n=38) were overweight or mildly obese, and the control group might have been consuming an excessive amount of protein that consisted of more than 50% of their diet. On the contrary, mice in the control group were fed a diet that consisted of 21% protein.
In the same study, they fed C57BL/6J wild-type mice a diet with reduced branched-chain amino acids (BCAAs) and found it was sufficient to improve metabolic health markers and body composition and had equivalent health effects to those of total PR.
Animal studies
Studies in rodents on the effect of PR consistently show that reduced protein intake, independently of total calorie consumption, leads to a variety of improved metabolic health parameters, as well as increased food intake and energy expenditure.[1] Some scientists argue that PR has wider health benefits over traditional CR, which derive from the additional energy expenditure observed in PR diets.[21][22][23]
Mice
In C57BL/6 mice of both sexes fed ad libitum, 5% dietary protein during 14 months led to increased food intake, increased adiposity, improved gluocose tolerance, reduced circulating IGF-1 and reduced mTOR activation in comparison to mice fed 60% protein.[24] Researchers argued that the benefits of caloric restriction are maximized when controlling for the ratio of macronutrients rather than total caloric intake.[24] Another study looked at male C57BL/6 mice fed 5% protein during a timeframe of only 14 days, and found that besides increased food intake and energy expenditure, there were increased levels of circulating FGF21 and increased expression of eIF2α in the liver, supporting the current understanding of the underlying biological mechanism.[21]
A range of other PR studies in C57BL/6 mice ranging from 4-7% of total protein in diet during intervals of 12-27 weeks showed similar positive outcomes and, additionally, recorded weight loss, reduced fat mass gain, reduced lean mass, improved glucose and pyruvate tolerance and improved expression of health markers in BAT (brown adipose tissue) and WAT (white adipose tissue).[7][25][26][27][28][29]
Several different studies have found that PR in the 5-7% range increases the lifespan of C57BL/6J male mice [3][30], and this effect has been shown to require FGF21.[31]
Rats
Sprague-Dawley male rats fed ad libitum with 9% of protein in diets during 14 days, led to increased food intake and increased levels of FGF21 and eIF2α in the liver, similarly to mice.[21] In another study of Sprague-Dawley male rats with 10% dietary protein intake during 14 days, increased hepatic autophagy and reduced hepatic lipogenic expression was observed.[32]
However, obesity-prone rats with completely abolished protein intake (0%), showed decreased energy intake and induced fatty liver which persisted after the restriction period, which can be associated to poor health.[33] In the same study, energy intake was increased and there was no incidence of fatty liver in rats that were fed 5% protein compared to 15% protein in the control diet.[33] This study shows that moderate, but not complete, protein restriction might be beneficial to health.
Additionally, in Wistar fatty rats, an animal model of type 2 diabetes and obesity, low-protein diets demonstrated a health benefit in diabetic status and prevented diabetic nephropathy.[34]
Caveats
Together with a range of health benefits, caloric restriction (CR) is often accompanied by a number of important caveats.[35] Some of the deleterious effects of CR include reduced bone density,[36] impaired wound healing[37] and an increased risk of infection.[38]
Similarly, recent studies have shown that protein restriction (PR) can increase frailty and shorten the lifespan of animals when PR is performed under stressful conditions.[39] Moderate protein restriction in Drosophila fruit flies led to lifespan extension at benign temperatures of 25-27ºC, but there was no effect or even a shortening of lifespan in cold (21-23ºC) or hot (29ºC) temperatures.[39]
- ↑ Jump up to: 1.0 1.1 Green CL, Lamming DW. Regulation of metabolic health by essential dietary amino acids. Mech Ageing Dev. 2019 Jan;177:186-200. doi:10.1016/j.mad.2018.07.004. [Epub 2018 Jul 22]. PMID: 30044947; PMCID: PMC6333505
- ↑ Mair, W., Piper, M., & Partridge, L. (2005). Calories Do Not Explain Extension of Life Span by Dietary Restriction in Drosophila. Plos Biology, 3(7), e223. doi: 10.1371/journal.pbio.0030223
- ↑ Jump up to: 3.0 3.1 Solon-Biet SM, McMahon AC, Ballard JW, Ruohonen K, Wu LE, Cogger VC, Warren A, Huang X, Pichaud N, Melvin RG, Gokarn R, Khalil M, Turner N, Cooney GJ, Sinclair DA, Raubenheimer D, Le Couteur DG, Simpson SJ. The ratio of macronutrients, not caloric intake, dictates cardiometabolic health, aging, and longevity in ad libitum-fed mice. Cell Metab. 2014 Mar 4; 19(3):418-30. doi: 10.1016/j.cmet.2014.02.009.
- ↑ Green, C., & Lamming, D. (2019). Regulation of metabolic health by essential dietary amino acids. Mechanisms Of Ageing And Development, 177, 186-200. doi: 10.1016/j.mad.2018.07.004
- ↑ Jump up to: 5.0 5.1 Laeger, T., Henagan, T., Albarado, D., Redman, L., Bray, G., Noland, R., Münzberg, H., Hutson, S., Gettys, T., Schwartz, M. and Morrison, C., 2014. FGF21 is an endocrine signal of protein restriction. Journal of Clinical Investigation, 124(9), pp.3913-3922.
- ↑ De Sousa-Coelho, A., Marrero, P., & Haro, D. (2012). Activating transcription factor 4-dependent induction of FGF21 during amino acid deprivation. Biochemical Journal, 443(1), 165-171. doi: 10.1042/bj20111748
- ↑ Jump up to: 7.0 7.1 7.2 Fontana, L., Cummings, N., Arriola Apelo, S., Neuman, J., Kasza, I., & Schmidt, B. et al. (2016). Decreased Consumption of Branched-Chain Amino Acids Improves Metabolic Health. Cell Reports, 16(2), 520-530. doi: 10.1016/j.celrep.2016.05.092
- ↑ Hill, C. and Kaeberlein, M., 2021. Anti-ageing effects of protein restriction unpacked. Nature, 589(7842), pp.357-358.
- ↑ Lu, J., Temp, U., Müller-Hartmann, A., Esser, J., Grönke, S. and Partridge, L., 2020. Sestrin is a key regulator of stem cell function and lifespan in response to dietary amino acids. Nature Aging, 1(1), pp.60-72.
- ↑ Miller, R., Buehner, G., Chang, Y., Harper, J., Sigler, R., & Smith-Wheelock, M. (2005). Methionine-deficient diet extends mouse lifespan, slows immune and lens aging, alters glucose, T4, IGF-I and insulin levels, and increases hepatocyte MIF levels and stress resistance. Aging Cell, 4(3), 119-125. doi: 10.1111/j.1474-9726.2005.00152.x
- ↑ Forney, L., Wanders, D., Stone, K., Pierse, A., & Gettys, T. (2017). Concentration-dependent linkage of dietary methionine restriction to the components of its metabolic phenotype. Obesity, 25(4), 730-738. doi: 10.1002/oby.21806
- ↑ Nichenametla, S., Mattocks, D., Malloy, V., & Pinto, J. (2018). Sulfur amino acid restriction-induced changes in redox-sensitive proteins are associated with slow protein synthesis rates. Annals Of The New York Academy Of Sciences, 1418(1), 80-94. doi: 10.1111/nyas.13556
- ↑ Douris, N., Stevanovic, D., Fisher, f., Cisu, T., Chee, M., & Nguyen, N. et al. (2015). Central Fibroblast Growth Factor 21 Browns White Fat via Sympathetic Action in Male Mice. Endocrinology, 156(7), 2470-2481. doi: 10.1210/en.2014-2001
- ↑ Yu, D., Yang, S., Miller, B., Wisinski, J., Sherman, D., & Brinkman, J. et al. (2018). Short‐term methionine deprivation improves metabolic health via sexually dimorphic, mTORCI‐independent mechanisms. The FASEB Journal, 32(6), 3471-3482. doi: 10.1096/fj.201701211r
- ↑ Levine, M., Suarez, J., Brandhorst, S., Balasubramanian, P., Cheng, C., & Madia, F. et al. (2014). Low Protein Intake Is Associated with a Major Reduction in IGF-1, Cancer, and Overall Mortality in the 65 and Younger but Not Older Population. Cell Metabolism, 19(3), 407-417. doi: 10.1016/j.cmet.2014.02.006
- ↑ Sun, Q. (2012). Red Meat Consumption and Mortality. Archives Of Internal Medicine, 172(7), 555. doi: 10.1001/archinternmed.2011.2287
- ↑ Grimberg, A. (2003). Mechanisms by which IGF-I May Promote Cancer. Cancer Biology &Amp; Therapy, 2(6), 628-633. doi: 10.4161/cbt.2.6.678
- ↑ Hua, H., Kong, Q., Yin, J., Zhang, J., & Jiang, Y. (2020). Insulin-like growth factor receptor signaling in tumorigenesis and drug resistance: a challenge for cancer therapy. Journal Of Hematology &Amp; Oncology, 13(1). doi: 10.1186/s13045-020-00904-3
- ↑ Pollak, M., Schernhammer, E., & Hankinson, S. (2004). Insulin-like growth factors and neoplasia. Nature Reviews Cancer, 4(7), 505-518. doi: 10.1038/nrc1387
- ↑ Sluijs, I., Beulens, J., van der A, D., Spijkerman, A., Grobbee, D., & van der Schouw, Y. (2009). Dietary Intake of Total, Animal, and Vegetable Protein and Risk of Type 2 Diabetes in the European Prospective Investigation into Cancer and Nutrition (EPIC)-NL Study. Diabetes Care, 33(1), 43-48. doi: 10.2337/dc09-1321
- ↑ Jump up to: 21.0 21.1 21.2 Laeger, T., Henagan, T., Albarado, D., Redman, L., Bray, G., & Noland, R. et al. (2014). FGF21 is an endocrine signal of protein restriction. Journal Of Clinical Investigation, 124(9), 3913-3922. doi: 10.1172/jci74915
- ↑ Morrison, C., Xi, X., White, C., Ye, J., & Martin, R. (2007). Amino acids inhibit Agrp gene expression via an mTOR-dependent mechanism. American Journal Of Physiology-Endocrinology And Metabolism, 293(1), E165-E171. doi: 10.1152/ajpendo.00675.2006
- ↑ White, P., Lapworth, A., An, J., Wang, L., McGarrah, R., & Stevens, R. et al. (2016). Branched-chain amino acid restriction in Zucker-fatty rats improves muscle insulin sensitivity by enhancing efficiency of fatty acid oxidation and acyl-glycine export. Molecular Metabolism, 5(7), 538-551. doi: 10.1016/j.molmet.2016.04.006
- ↑ Jump up to: 24.0 24.1 Solon-Biet, S., McMahon, A., Ballard, J., Ruohonen, K., Wu, L., & Cogger, V. et al. (2014). The Ratio of Macronutrients, Not Caloric Intake, Dictates Cardiometabolic Health, Aging, and Longevity in Ad Libitum-Fed Mice. Cell Metabolism, 19(3), 418-430. doi: 10.1016/j.cmet.2014.02.009
- ↑ Laeger, T., Albarado, D., Burke, S., Trosclair, L., Hedgepeth, J., & Berthoud, H. et al. (2016). Metabolic Responses to Dietary Protein Restriction Require an Increase in FGF21 that Is Delayed by the Absence of GCN2. Cell Reports, 16(3), 707-716. doi: 10.1016/j.celrep.2016.06.044
- ↑ Maida, A., Zota, A., Sjøberg, K., Schumacher, J., Sijmonsma, T., & Pfenninger, A. et al. (2016). A liver stress-endocrine nexus promotes metabolic integrity during dietary protein dilution. Journal Of Clinical Investigation, 126(9), 3263-3278. doi: 10.1172/jci85946
- ↑ Solon-Biet, S., Cogger, V., Pulpitel, T., Heblinski, M., Wahl, D., & McMahon, A. et al. (2016). Defining the Nutritional and Metabolic Context of FGF21 Using the Geometric Framework. Cell Metabolism, 24(4), 555-565. doi: 10.1016/j.cmet.2016.09.001
- ↑ Hill, C., Laeger, T., Albarado, D., McDougal, D., Berthoud, H., Münzberg, H., & Morrison, C. (2017). Low protein-induced increases in FGF21 drive UCP1-dependent metabolic but not thermoregulatory endpoints. Scientific Reports, 7(1). doi: 10.1038/s41598-017-07498-w
- ↑ Cummings, N., Williams, E., Kasza, I., Konon, E., Schaid, M., & Schmidt, B. et al. (2017). Restoration of metabolic health by decreased consumption of branched-chain amino acids. The Journal Of Physiology, 596(4), 623-645. doi: 10.1113/jp275075
- ↑ Richardson NE, Konon EN, Schuster HS, Mitchell AT, Boyle C, Rodgers AC, Finke M, Haider LR, Yu D, Flores V, Pak HH, Ahmad S, Ahmed S, Radcliff A, Wu J, Williams EM, Abdi L, Sherman DS, Hacker T, Lamming DW. Lifelong restriction of dietary branched-chain amino acids has sex-specific benefits for frailty and lifespan in mice. Nature Aging, 2021 Jan;1(1):73-86. doi: 10.1038/s43587-020-00006-2. Epub 2021 Jan 14. PMID: 33796866 PMCID: PMC8009080.
- ↑ Hill CM, Albarado DC, Coco LG, Spann RA, Khan MS, Qualls-Creekmore E, Burk DH, Burke SJ, Collier JJ, Yu S, McDougal DH, Berthoud HR, Münzberg H, Bartke A, Morrison CD. FGF21 is required for protein restriction to extend lifespan and improve metabolic health in male mice. Nature Communications, 2022 Apr 7;13(1):1897. doi: 10.1038/s41467-022-29499-8. PMID: 35393401. PMCID: PMC8991228.
- ↑ Henagan, T., Laeger, T., Navard, A., Albarado, D., Noland, R., & Stadler, K. et al. (2016). Hepatic autophagy contributes to the metabolic response to dietary protein restriction. Metabolism, 65(6), 805-815. doi: 10.1016/j.metabol.2016.02.015
- ↑ Jump up to: 33.0 33.1 Pezeshki, A., Zapata, R., Singh, A., Yee, N., & Chelikani, P. (2016). Low protein diets produce divergent effects on energy balance. Scientific Reports, 6(1). doi: 10.1038/srep25145
- ↑ Kitada, M., Ogura, Y., Suzuki, T., Monno, I., Kanasaki, K., Watanabe, A., & Koya, D. (2018). A low-protein diet exerts a beneficial effect on diabetic status and prevents diabetic nephropathy in Wistar fatty rats, an animal model of type 2 diabetes and obesity. Nutrition &Amp; Metabolism, 15(1). doi: 10.1186/s12986-018-0255-1
- ↑ Ingram, D., & de Cabo, R. (2017). Calorie restriction in rodents: Caveats to consider. Ageing Research Reviews, 39, 15-28. doi: 10.1016/j.arr.2017.05.008
- ↑ Baek, K., Barlow, A., Allen, M., & Bloomfield, S. (2008). Food restriction and simulated microgravity: effects on bone and serum leptin. Journal Of Applied Physiology, 104(4), 1086-1093. doi: 10.1152/japplphysiol.01209.2007
- ↑ Hunt, N., Li, G., Zhu, M., Levette, A., Chachich, M., & Spangler, E. et al. (2011). Effect of calorie restriction and refeeding on skin wound healing in the rat. AGE, 34(6), 1453-1458. doi: 10.1007/s11357-011-9321-6
- ↑ Kristan, D. (2008). Calorie restriction and susceptibility to intact pathogens. AGE, 30(2-3), 147-156. doi: 10.1007/s11357-008-9056-1
- ↑ Jump up to: 39.0 39.1 Zajitschek, Felix, et al. “Dietary Restriction Fails to Extend Life in Stressful Environments.” 2022, https://doi.org/10.1101/2022.10.17.512576.