CD38
CD38 is a cell surface marker (cluster of differentiation 38) identified as a 45 kDa 300-amino-acid glycoprotein encoded by the CD38 human gene which is located on band p15 of chromosome four as a single copy gene. [1][2]
CD38 activities in the immune system
CD38 is involved in activation and proliferation of T cells, differentiation of B cell, and chemotaxis of neutrophils, monocytes and some other immune cells capable of infiltrating organs and tissues. [3][4]
CD38-deficient mice show increased susceptibility to bacterial infections due to a weakened immune system. [5]
CD38 plays crucial roles in calcium signaling
CD38 is a single-pass transmembrane enzyme catalyzing basically the synthesis of two nucleotide second messengers, cyclic ADP-ribose (cADPR) from NAD+ and nicotinic acid adenine dinucleotide phosphate (NAADP) from NADP. [6]
CD38 exists in two opposite membrane orientations. If its catalytic domain facing the cytosol, it is responsible for producing cellular cADPR that mediates the mobilization of the endoplasmic Ca2+ -stores in response to a wide range of stimuli[6]. In an opposite orientation, it is a surface receptor mediating extracellular functions such as immune cell adhesion, activation and proliferation, as well as the release of pro-inflammatory and regulatory cytokines. [7] At an acidic pH, surface CD38 produces yet another Ca2+-messenger, NAADP, when delivered to the endo-lysosomes by induced endocytosis. [6] [8]
Soluble CD38
Soluble extracellular domain of CD38 is detected in biological fluids due to solubilization by endopeptidase in certain conditions such as inflammation caused by lipopolysaccharides from damaged cell walls[9]. Lipopolysaccharide could also upregulate the expression of CD38 in M1 macrophages which are immune effector cells that resist bacterial invasion and phagocytizes and digest necrotic cells[9]
CD38 affects processes of bone formation and resorption in response to mechanical stress
CD38 knockout mice displayed bone loss with increased osteoclast levels and reduced bone mineral densities similar to that was seen in osteoporosis. [10]
Skeletal mechanical weight unloading (such as caused by prolonged bed rest or sedentary life) reduces CD38 expression that recovers to normal levels after reloading. [11]
CD38 as a metabolic sensor and regulator
An important role in various cellular processes, including metabolism, cellular signaling, epigenetics and DNA repair plays NAD+ (Nicotinamide adenine dinucleotide) a critical redox coenzyme. [12] [13][14] By modulating NAD+-sensing enzymes (such as Sirtuins), NAD+ controls hundreds of key processes from energy metabolism to cell survival. [14]
NAD+ levels steadily decline with age, resulting in altered metabolism and increased disease susceptibility. [12][13] [14] It was shown that the enzyme CD38 is one of the main NAD-degrading enzymes in mammalian tissues.[13][4] In addition to NAD+, CD38 metabolizes extracellular NAD+ precursors Nicotinamide mononucleotide (NMN) and Nicotinamide riboside (NR) prior to their transport into the cell for NAD+ biosynthesis.[13]
It was discovered that inflammatory cytokines secreted by senescent cells (the senescence-associated secretory phenotype, SASP) induce pro-inflammatory M1-like macrophages to proliferate and express CD38. [15] [16] This highlights senescent cells as an important factor in NAD+ decline because the accumulation of senescent cells with age leads to increased systemic secretion of the pro-inflammatory SASP, which in turn leads to elevation of cellular CD38 expression and a concurrent decrease in NAD+ levels. NAD+ restoration has been identified as a key therapeutic target that can positively impact many of the hallmarks of cellular aging.[17] Boosting NAD+ via genetic or pharmacological CD38 targeting or NAD+ precursor supplementation protected mice from skin, lung, and peritoneal fibrosis. [18] Long-living-individuals (>95 years), carriers (both hetero and homozygous) of longevity-associated variant LAV-BPIFB4 gene displayed significantly higher NAD+ circulating level, when compared with no-carriers, apparently due to reduction of the frequency of CD38+ immune cells[19] NAD+ repletion with the NAD+ precursor nicotinamide riboside (NR) improved mitochondrial and stem cell function and enhanced life span in mice.[20] However, increasing NAD+ using a precursor without first inhibiting CD38 may ‘fuel’ CD38-mediated inflammation due to increased secretion of pro-inflammatory tumorigenic SASP cytokines leading to elevation of cellular CD38 expression and so to repeat decrease in NAD+ levels.[21] Thus, dietary NAD+ augmenting supplement should be administered with precision to balance the advantageous anti-ageing effects with potential detrimental pro-tumorigenic side effects.[22]
CD38 inhibitors
Since CD38 plays a central role in reducing the NAD+ pool, artificially maintaining a high level of NAD+ by inhibiting CD38 may have a positive effect on metabolic diseases and the aging.[23][24] Since CD38 must degrade nearly 100 molecules of NAD to generate one molecule of cADPR,[25] strategies to inhibit CD38 even at a low level may lead to substantial increases in cellular NAD+ levels.[26]
The ability to inhibit CD38 have such substances as: Flavonoids[27] (in particular apigenin.[28] The reason for which it is useful to eat celery (Apium graveolens), parsley and radish leaves - they are source of apigenin.[29]).
Anthocyanins, such as luteolinidin, protects endothelial and myocardial function in the postischemic heart through CD38 inhibition.[30]
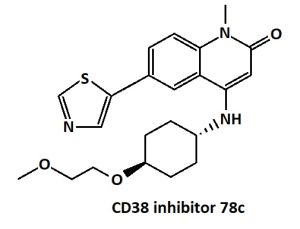
In diseases associated with aging, for therapy that increases NAD+ can be used a 78c inhibitor.[31][32] For example, 78c increased the level of NAD+ in the liver by 536% just two hours after ingestion.[33] Treatment of old mice with the NADase 78c inhibitor markedly reduced the accumulation of inflammatory cells in tissues and significantly reduced the appearance of fibrotic and inflammatory changes with aging in muscles [32] , as well as in the skin, lungs and peritoneal mucosa.[18] In in vitro experiments on mouse hearts, treatment with 78c significantly reduced the effects of myocardial infarction.[34]
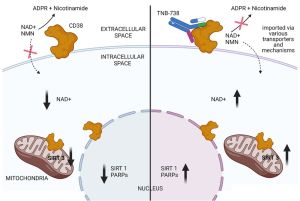
78c has been shown to increase lifespan (average by 17% and maximal by 14%) and protect against aging-induced health loss in aged male mice.[35]
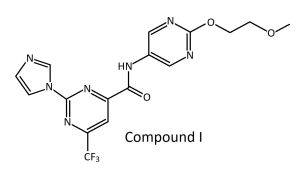
Orally bioavailable enzymatic inhibitor of CD38, MK-0159 in experiments with mice show strong protection from myocardial damage upon cardiac ischemia/reperfusion injury in the murine heart.[37] Administration of the CD38 inhibitor MK-0159 reverses mitochondrial defects and restores CD8+ T cells function against infections in mice and so substantially improves the pathology linked to viral infection.[38]
The potential to treat various diseases associated with NAD+ deficiency without the potential side effects of small molecules and cytotoxic antibodies has an IgG (immunoglobulin) antibody TNB-738 that neither crosses the cell membrane nor the blood–brain barrier. The desired mechanism of action of TNB-738 is strictly enzyme inhibition without CD38+ cell depletion, thus avoiding side-effects associated with the lysis of subpopulations of immune cells, such as monocytes, effector T cells, and NK cells.[39]
The promising candidate for its excellent in vivo efficacy, favorable pharmacokinetics, and attractive safety profile, called compound 1 has a great effect on mitochondrial function, metabolic processes, muscle contraction/development, and actin filament organization via regulating the expression of relevant genes associated with an elevated NAD+ level.[36]
References
- ↑ Nakagawara, K., Mori, M., Takasawa, S., Nata, K., Takamura, T., Berlova, A., ... & Okamoto, H. (1995). Assignment of CD38, the gene encoding human leukocyte antigen CD38 (ADP-ribosyl cyclase/cyclic ADP-ribose hydrolase), to chromosome 4p15. Cytogenetic and Genome Research, 69(1-2), 38-39. PMID:7835083 DOI:10.1159/000133933
- ↑ Nata, K., Takamura, T., Karasawa, T., Kumagai, T., Hashioka, W., Tohgo, A., ... & Okamoto, H. (1997). Human gene encoding CD38 (ADP-ribosyl cyclase/cyclic ADP-ribose hydrolase): organization, nucleotide sequence and alternative splicing. Gene, 186(2), 285-292. PMID:9074508 DOI:10.1016/s0378-1119(96)00723-8
- ↑ Takasawa, S. (2022). CD38–Cyclic ADP-Ribose Signal System in Physiology, Biochemistry, and Pathophysiology. International Journal of Molecular Sciences, 23(8), 4306. PMID: 35457121 PMC9033130 DOI: 10.3390/ijms23084306
- ↑ 4.0 4.1 Zeidler, J. D., Hogan, K. A., Agorrody, G., Peclat, T. R., Kashyap, S., Kanamori, K. S., ... & Chini, E. N. (2022). The CD38 glycohydrolase and the NAD sink: implications for pathological conditions. American Journal of Physiology-Cell Physiology, 322(3), C521-C545 PMID: 35138178 PMC8917930 (available on 2023-03-01) DOI: 10.1152/ajpcell.00451.2021
- ↑ Glaría, E., & Valledor, A. F. (2020). Roles of CD38 in the Immune Response to Infection. Cells, 9(1), 228. PMID: 31963337 PMC7017097 DOI: 10.3390/cells9010228
- ↑ 6.0 6.1 6.2 Lee, H. C., Deng, Q. W., & Zhao, Y. J. (2022). The calcium signaling enzyme CD38-a paradigm for membrane topology defining distinct protein functions. Cell Calcium, 101, 102514. PMID:34896700 DOI:10.1016/j.ceca.2021.102514
- ↑ Astigiano, C., Benzi, A., Laugieri, M. E., Piacente, F., Sturla, L., Guida, L., ... & De Flora, A. (2022). Paracrine ADP Ribosyl Cyclase-Mediated Regulation of Biological Processes. Cells, 11(17), 2637. PMID:36078044 PMC9454491 DOI: 10.3390/cells11172637
- ↑ Wo, Y. J., Gan, A. S. P., Lim, X., Tay, I. S. Y., Lim, S., Lim, J. C. T., & Yeong, J. P. S. (2019). The roles of CD38 and CD157 in the solid tumor microenvironment and cancer immunotherapy. Cells, 9(1), 26. PMID: 31861847 PMC7017359 DOI: 10.3390/cells9010026
- ↑ 9.0 9.1 Lee, C. U., Song, E. K., Yoo, C. H., Kwak, Y. K., & Han, M. K. (2012). Lipopolysaccharide induces CD38 expression and solubilization in J774 macrophage cells. Molecules and cells, 34(6), 573-576. PMID: 30975470 PMCID: PMC6486859 DOI: 10.1016/j.bbrc.2019.03.199
- ↑ Sun, L., Iqbal, J., Dolgilevich, S., Yuen, T., Wu, X. B., Moonga, B. S., ... & Zaidi, M. (2003). Disordered osteoclast formation and function in a CD38 (ADP‐ribosyl cyclase) ‐deficient mouse establishes an essential role for CD38 in bone resorption. The FASEB journal, 17(3), 369-375. PMID: 12631576 DOI: 10.1096/fj.02-0205com
- ↑ Moridera, K., Uchida, S., Tanaka, S., Menuki, K., Utsunomiya, H., Yamaoka, K., ... & Sakai, A. (2020). Skeletal unloading reduces cluster of differentiation (CD) 38 expression in the bone marrow and osteoblasts of mice. Journal of Orthopaedic Science, 25(2), 331-337. PMID: 31072650 DOI: 10.1016/j.jos.2019.03.023
- ↑ 12.0 12.1 Chini, C. C., Tarragó, M. G., & Chini, E. N. (2017). NAD and the aging process: Role in life, death and everything in between. Molecular and cellular endocrinology, 455, 62-74. PMID: 27825999 PMC5419884 doi:[https://doi.org/10.1016/j.mce.2016.11.003
- ↑ 13.0 13.1 13.2 13.3 Camacho-Pereira, J., Tarragó, M. G., Chini, C. C., Nin, V., Escande, C., Warner, G. M., ... & Chini, E. N. (2016). CD38 dictates age-related NAD decline and mitochondrial dysfunction through an SIRT3-dependent mechanism. Cell metabolism, 23(6), 1127-1139. PMID: 27304511 PMC4911708 DOI:10.1016/j.cmet.2016.05.006
- ↑ 14.0 14.1 14.2 Rajman, L., Chwalek, K., & Sinclair, D. A. (2018). Therapeutic potential of NAD-boosting molecules: the in vivo evidence. Cell metabolism, 27(3), 529-547. PMID: 29514064 PMC6342515 DOI:10.1016/j.cmet.2018.02.011
- ↑ Covarrubias, A. J., Kale, A., Perrone, R., Lopez-Dominguez, J. A., Pisco, A. O., Kasler, H. G., ... & Verdin, E. (2020). Senescent cells promote tissue NAD+ decline during ageing via the activation of CD38+ macrophages. Nature metabolism, 2(11), 1265-1283. PMID: 33199924 PMC7908681 DOI:10.1038/s42255-020-00305-3
- ↑ Chini, C., Peclat, T. R., Warner, G. M., Kashyap, S., Espindola-Netto, J. M., de Oliveira, G. C., ... & Chini, E. N. (2020). CD38 ecto-enzyme in immune cells is induced during aging and regulates NAD+ and NMN levels. Nature metabolism, 2(11), 1284-1304. PMID: 33199925 PMC8752031 DOI:10.1038/s42255-020-00298-z
- ↑ Conlon, N. J. (2022). The Role of NAD+ in Regenerative Medicine. Plastic and reconstructive surgery, 150, 41S-48S. PMID:36170435 PMC9512238 DOI:10.1097/PRS.0000000000009673
- ↑ 18.0 18.1 Shi, B., Wang, W., Korman, B., Kai, L., Wang, Q., Wei, J., ... & Varga, J. (2021). Targeting CD38-dependent NAD+ metabolism to mitigate multiple organ fibrosis. Iscience, 24(1), 101902. PMID: 33385109 PMC7770554 DOI:10.1016/j.isci.2020.101902
- ↑ Ciaglia, E., Lopardo, V., Montella, F., Carrizzo, A., Di Pietro, P., Malavolta, M., ... & Puca, A. A. (2022). "Transfer of the longevity-associated variant of BPIFB4 gene rejuvenates immune system and vasculature by a reduction of CD38+ macrophages and NAD+ decline". Cell death & disease, 13(1), 1-10. PMID: 35087020 PMC8792139 DOI:10.1038/s41419-022-04535-z
- ↑ Zhang, H., Ryu, D., Wu, Y., Gariani, K., Wang, X., Luan, P., ... & Auwerx, J. (2016). NAD+ repletion improves mitochondrial and stem cell function and enhances life span in mice. Science, 352(6292), 1436-1443. PMID: 27127236 DOI:10.1126/science.aaf2693
- ↑ Nacarelli, T., Lau, L., Fukumoto, T., Zundell, J., Fatkhutdinov, N., Wu, S., ... & Zhang, R. (2019). NAD+ metabolism governs the proinflammatory senescence-associated secretome. Nature cell biology, 21(3), 397-407. PMID: 30778219 PMC6448588 DOI:10.1038/s41556-019-0287-4
- ↑ Maric, T., Bazhin, A., Khodakivskyi, P., Mikhaylov, G., Solodnikova, E., Yevtodiyenko, A., ... & Goun, E. (2023). A bioluminescent-based probe for in vivo non-invasive monitoring of nicotinamide riboside uptake reveals a link between metastasis and NAD+ metabolism. Biosensors and Bioelectronics, 220, 114826. PMID: 36371959 DOI:10.1016/j.bios.2022.114826
- ↑ Tarragó, M. G., Chini, C. C., Kanamori, K. S., Warner, G. M., Caride, A., de Oliveira, G. C., ... & Chini, E. N. (2018). A potent and specific CD38 inhibitor ameliorates age-related metabolic dysfunction by reversing tissue NAD+ decline. Cell metabolism, 27(5), 1081-1095. PMID:29719225 PMC:5935140 doi:10.1016/j.cmet.2018.03.016
- ↑ Peclat, T. R., Thompson, K. L., Warner, G. M., Chini, C. C., Tarragó, M. G., Mazdeh, D. Z., ... & Chini, E. N. (2022). CD38 inhibitor 78c increases mice lifespan and healthspan in a model of chronological aging. Aging Cell, e13589. PMID:35263032 doi:10.1111/acel.13589
- ↑ Chini, E. N. (2009). CD38 as a regulator of cellular NAD: a novel potential pharmacological target for metabolic conditions. Current pharmaceutical design, 15(1), 57-63. PMID: 19149603 PMC2883294 DOI: 10.2174/138161209787185788
- ↑ Conlon, N., & Ford, D. (2022). A systems-approach to NAD+ restoration. Biochemical pharmacology, 114946. https://doi.org/10.1016/j.bcp.2022.114946
- ↑ Kellenberger, E., Kuhn, I., Schuber, F., & Muller-Steffner, H. (2011). Flavonoids as inhibitors of human CD38. Bioorganic & medicinal chemistry letters, 21(13), 3939-3942. PMID: 21641214 DOI: 10.1016/j.bmcl.2011.05.022
- ↑ Escande, C., Nin, V., Price, N. L., Capellini, V., Gomes, A. P., Barbosa, M. T., ... & Chini, E. N. (2013). Flavonoid apigenin is an inhibitor of the NAD+ ase CD38: implications for cellular NAD+ metabolism, protein acetylation, and treatment of metabolic syndrome. Diabetes, 62(4), 1084-1093.
- ↑ Li, Z., Zhou, J., Ji, L., Liang, Y., & Xie, S. (2022). Recent Advances in the Pharmacological Actions of Apigenin, Its Complexes, and Its Derivatives. Food Reviews International, 1-34. https://doi.org/10.1080/87559129.2022.2122989
- ↑ Boslett, J., Hemann, C., Zhao, Y. J., Lee, H. C., & Zweier, J. L. (2017). Luteolinidin protects the postischemic heart through CD38 inhibition with preservation of NAD (P)(H). Journal of Pharmacology and Experimental Therapeutics, 361(1), 99-108. PMID: 28108596 PMC5363772 DOI: 10.1124/jpet.116.239459
- ↑ Chini, E. N., Chini, C. C., Netto, J. M. E., de Oliveira, G. C., & van Schooten, W. (2018). The pharmacology of CD38/NADase: an emerging target in cancer and diseases of aging. Trends in pharmacological sciences, 39(4), 424-436. PMID:29482842 PMC5885288 doi:10.1016/j.tips.2018.02.001
- ↑ 32.0 32.1 Tarragó, M. G., Chini, C. C., Kanamori, K. S., Warner, G. M., Caride, A., de Oliveira, G. C., ... & Chini, E. N. (2018). A potent and specific CD38 inhibitor ameliorates age-related metabolic dysfunction by reversing tissue NAD+ decline. Cell metabolism, 27(5), 1081-1095. PMID:29719225 PMC5935140 doi:10.1016/j.cmet.2018.03.016
- ↑ Haffner, C. D., Becherer, J. D., Boros, E. E., Cadilla, R., Carpenter, T., Cowan, D., ... & Ulrich, J. C. (2015). Discovery, synthesis, and biological evaluation of thiazoloquin (az) olin (on) es as potent CD38 inhibitors. Journal of medicinal chemistry, 58(8), 3548-3571. PMID 25828863 doi:10.1021/jm502009h
- ↑ Boslett, J., Reddy, N., Alzarie, Y. A., & Zweier, J. L. (2019). Inhibition of CD38 with the Thiazoloquin (az) olin (on) e 78c Protects the Heart against Postischemic Injury. Journal of Pharmacology and Experimental Therapeutics, 369(1), 55-64. PMID 30635470 PMC6413770 doi:10.1124/jpet.118.254557
- ↑ Peclat, T. R., Thompson, K. L., Warner, G. M., Chini, C. C., Tarragó, M. G., Mazdeh, D. Z., ... & Chini, E. N. (2022). CD38 inhibitor 78c increases mice lifespan and healthspan in a model of chronological aging. Aging Cell, e13589. PMID:35263032 doi:10.1111/acel.13589
- ↑ 36.0 36.1 Li, Y., Liu, Y., Zhang, Y., Wu, Y., Xing, Z., Wang, J., & Fan, G. H. (2023). Discovery of a First-in-Class CD38 Inhibitor for the Treatment of Mitochondrial Myopathy. Journal of Medicinal Chemistry. PMID:37696000 DOI:10.1021/acs.jmedchem.3c00391
- ↑ Lagu, B., Wu, X., Kulkarni, S., Paul, R., Becherer, J. D., Olson, L., ... & Andrzejewski, S. (2022). Orally Bioavailable Enzymatic Inhibitor of CD38, MK-0159, Protects against Ischemia/Reperfusion Injury in the Murine Heart. Journal of medicinal chemistry, 65(13), 9418-9446. PMID:35762533 DOI:10.1021/acs.jmedchem.2c00688
- ↑ Chen, P. M., Katsuyama, E., Satyam, A., Li, H., Rubio, J., Jung, S., ... & Tsokos, G. C. (2022). CD38 reduces mitochondrial fitness and cytotoxic T cell response against viral infection in lupus patients by suppressing mitophagy. Science Advances, 8(24), eabo4271. PMID: 35704572 PMC9200274 DOI: 10.1126/sciadv.abo4271
- ↑ Ugamraj, H. S., Dang, K., Ouisse, L. H., Buelow, B., Chini, E. N., Castello, G., ... & Dalvi, P. (2022). TNB-738, a biparatopic antibody, boosts intracellular NAD+ by inhibiting CD38 ecto-enzyme activity. MAbs, 14(1), 2095949 PMID:35867844 PMC9311320 DOI:10.1080/19420862.2022.2095949