CISD2
CISD2 (CDGSH Iron Sulfur Domain 2) is an evolutionarily conserved pro-longevity gene (also known as: ERIS (endoplasmic reticulum intermembrane small protein), Miner1, NAF-1 (nutrient-deprivation autophagy factor-1), WFS2, and ZCD2) that is located at position 24 on the long arm of human chromosome 4 (4q24) – the region significantly contributing to life-span control as has been revealed by a genome-wide linkage scan of long-lived families. [1][2] CISD2 localizes onto the endoplasmic reticulum (ER), the outer mitochondrial membrane and the mitochondria-associated membrane. It plays a crucial role in the regulation of cytosolic Ca2+ homeostasis, ER integrity and in preventing mitochondrial dysfunction, and also in the activation of autophagy and apoptosis in different cells. [3] [4][5][6] By functioning as a transport conduit across intracellular membranes for labile iron and calcium, CISD2 protects cells from overaccumulation of iron and calcium excitotoxicity.[7]
A disruption in CISD2 cluster transfer activity (via inducible expression of a point mutant of CISD2 (H114C) with a significantly higher stability of the CISD2 [2Fe-2S] cluster) results in the activation of oxidative stress that triggers a ferroptosis-like process of cell death. [8][9]
Elevated expression of NAF-1 is associated with the progression of multiple cancer types since CISD2 promotes rapid cell proliferation by preventing unstable mitochondrial labile iron (mLI) and reactive oxygen species (mROS) overloads.[10] NAF-1 protein are critical for inducing oxidative stress tolerance in cancer cells, leading to rapid tumor growth, and drugs that stabilize the NAF-1 cluster could be used as part of a treatment strategy for cancers that display high NAF-1 expression.[11][12]
The pre-mRNA of CISD2 has two splice sites, creating 3 exons and 2 introns. [13] CISD2 was identified as the carrier of a mutations that causes neurodegenerative diseases such as Wolfram Syndrome 2 (WFS2) and partly Alzheimer’s Disease due to alterations in the ER and mitochondrial Ca2+ homeostasis, mitochondrial dysfunction and increased ROS (Reactive oxygen species, chemically reactive molecules containing oxygen) production.[14] [15] CISD2, loss-of-function mutations in humans characterized by diabetes mellitus, optic atrophy, deafness peptic ulcer bleeding and defective platelet aggregation. [16][10] CISD2 knockout mice also show accelerated aging, blindness, an abnormal skeleton, and muscle atrophy, effects that are very similar to those described in the WFS2 patients.[10] [17]
Cisd2 level is a key determinant of lifespan and healthspan
Mouse Cisd2 deficiency shortens lifespan and accelerates aging that results in premature aging.[18]
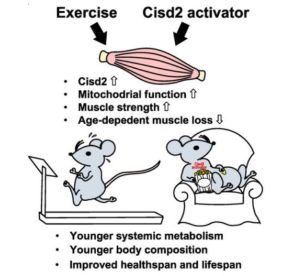
Cisd2 is essential to delaying cardiac aging and to maintaining heart functions. Cisd2 deficiency causes intercalated disc defects and leads to degeneration of the mitochondria and sarcomeres, thereby impairing its electromechanical functioning. Cisd2 deficiency also disrupts Ca2+ homeostasis via dysregulation of sarco/endoplasmic reticulum Ca2+-ATPase activity, resulting in an increased level of basal cytosolic Ca2+ and mitochondrial Ca2+ overload in cardiomyocytes.[20]
Conversely, a persistently high level of Cisd2 promotes longevity.[21] CISD2 protects cardiomyocytes from overaccumulation of iron, which is common in aging hearts and can contribute to the pathogenesis of heart failure.[22] Cisd2 overexpression modulates a number of aging-related pathways, namely the sirtuins signaling, autophagy, and senescence pathways, to bring about rejuvenation of the heart as it enters old age.[23] Cisd2 ameliorates age-associated degeneration of the skin, skeletal muscles and neurons. [21] Moreover, Cisd2 protects mitochondria from age-associated damage and functional decline as well as attenuating the age-associated reduction in whole-body energy metabolism. [21] It was shown that a persistent level of Cisd2 achieved by transgenic expression in mice extends their median and maximum lifespan without any apparent deleterious side effects.[7] According to Teng et al., exercise and Cisd2 activation are two very promising strategies as an individual ages to build a healthy lifespan.[19]
Natural compounds that can upregulate CISD2 expression
CISD2, the expression of which otherwise decreases during natural aging, can be pharmaceutically activated at a late-life stage of aged mice.[24] Treatment with dietary hesperetin, starting at 19–21 month old, has shown to enhance CISD2 gene expression and extend the lifespan and healthspan of mice. In addition, hesperidin and its aglycone, hesperetin treatment appears to attenuate whole-body metabolic decline, reducing fat and improving glucose homeostasis, as well as slowing down heart and skeletal muscle aging.[25][24] Hesperetin has various beneficial biological properties, which confer cardioprotective[26], anticancer,[27][28] antidiabetic effects,[29][30] protective effect against bone loss,[31] skin aging[32] and also against neurodegeneration triggered by aging.[30][33]
The beneficial anti-aging effects of hesperidin and its aglycone, hesperetin are largely dependent on CISD2 as revealed from transcriptomic analysis - most (79%) of the genes influenced by hesperetin lost their differential expression patterns in the absence of CISD2.[25][24] It would be interesting to check in the same way if the anti-aging effects of naringenin also depend on CISD2.[34]
Hesperetin is a well-known bioflavonoid that is found in dried peppermint leaves (60-200 mg/100 g),[35] as well as in numerous types of citrus fruits including- oranges, grapefruit, and tangerines upon ingestion, also in small amounts in sauerkraut.[36][37][30]
Inclusion complexes of hesperidin or hesperetin with hydroxypropyl β-cyclodextrin[38] or Soluplus®, alginate sodium, and hydroxypropylmethylcellulose in a 1:5 w/w ratio,[39] increase solubility and antioxidant potential. Such solid dispersions are promising delivery systems of hesperidin, and they can pose a more effective approach to treating civilization diseases.
The principal bright yellow compound obtained from the root of turmeric (Curcuma longa) named curcumin also was found to increase the CISD2 protein level in the astrocytes of the spinal cords of old mice.[40][41]
References
- ↑ Puca, A. A., Daly, M. J., Brewster, S. J., Matise, T. C., Barrett, J., Shea-Drinkwater, M., ... & Perls, T. (2001). A genome-wide scan for linkage to human exceptional longevity identifies a locus on chromosome 4. Proceedings of the National Academy of Sciences, 98(18), 10505-10508. PMID: 11526246 PMC56990 DOI: 10.1073/pnas.181337598
- ↑ Amr, S., Heisey, C., Zhang, M., Xia, X. J., Shows, K. H., Ajlouni, K., ... & Shiang, R. (2007). A homozygous mutation in a novel zinc-finger protein, ERIS, is responsible for Wolfram syndrome 2. The American Journal of Human Genetics, 81(4), 673-683.
- ↑ Shen, Z. Q., Chen, Y. F., Chen, J. R., Jou, Y. S., Wu, P. C., Kao, C. H., ... & Tsai, T. F. (2017). CISD2 haploinsufficiency disrupts calcium homeostasis, causes nonalcoholic fatty liver disease, and promotes hepatocellular carcinoma. Cell reports, 21(8), 2198-2211.
- ↑ Yeh, C. H., Chou, Y. J., Kao, C. H., & Tsai, T. F. (2020). Mitochondria and calcium homeostasis: Cisd2 as a big player in cardiac ageing. International Journal of Molecular Sciences, 21(23), 9238. PMID: 33287440 PMC7731030 DOI: 10.3390/ijms21239238
- ↑ Chen, Y. F., Kao, C. H., Kirby, R., & Tsai, T. F. (2009). Cisd2 mediates mitochondrial integrity and life span in mammals. Autophagy, 5(7), 1043-1045. DOI: 10.4161/auto.5.7.9351
- ↑ Chen, Y. F., Kao, C. H., Chen, Y. T., Wang, C. H., Wu, C. Y., Tsai, C. Y., ... & Tsai, T. F. (2009). Cisd2 deficiency drives premature aging and causes mitochondria-mediated defects in mice. Genes & development, 23(10), 1183-1194. PMID: 19451219 PMC2685531 DOI: 10.1101/gad.1779509
- ↑ 7.0 7.1 Shen, Z. Q., Huang, Y. L., Teng, Y. C., Wang, T. W., Kao, C. H., Yeh, C. H., & Tsai, T. F. (2021). CISD2 maintains cellular homeostasis. Biochimica et Biophysica Acta (BBA)-Molecular Cell Research, 1868(4), 118954. https://doi.org/10.1016/j.bbamcr.2021.118954
- ↑ Karmi, O., Sohn, Y. S., Zandalinas, S. I., Rowland, L., King, S. D., Nechushtai, R., & Mittler, R. (2021). Disrupting CISD2 function in cancer cells primarily impacts mitochondrial labile iron levels and triggers TXNIP expression. Free Radical Biology and Medicine, 176, 92-104.PMID: 34547371 PMC8761261 DOI: 10.1016/j.freeradbiomed.2021.09.013
- ↑ Zhang, M., Liu, Z., Le, Y., Gu, Z., & Zhao, H. (2022). Iron-Sulfur Clusters: A Key Factor of Regulated Cell Death in Cancer. Oxidative Medicine and Cellular Longevity, 2022. PMID: 36338346 PMC9629928 DOI: 10.1155/2022/7449941
- ↑ 10.0 10.1 10.2 Liao, H. Y., Liao, B., & Zhang, H. H. (2021). CISD2 plays a role in age-related diseases and cancer. Biomedicine & Pharmacotherapy, 138, 111472.
- ↑ Wang, L., Ouyang, F., Liu, X., Wu, S., Wu, H. M., Xu, Y., ... & Zhang, L. (2016). Overexpressed CISD2 has prognostic value in human gastric cancer and promotes gastric cancer cell proliferation and tumorigenesis via AKT signaling pathway. Oncotarget, 7(4), 3791. PMID: 26565812 PMC4826170 DOI: 10.18632/oncotarget.6302
- ↑ Wang, J., Hu, J., Wang, M., Yuan, H., Xing, Y., Zhou, X., ... & Zhu, L. (2022). CISD2 Promotes Proliferation of Colorectal Cancer Cells by Inhibiting Autophagy in a Wnt/β-Catenin-Signaling-Dependent Pathway. Biochemical Genetics, 1-13. PMID: 36008699 DOI: 10.1007/s10528-022-10267-8
- ↑ Conlan, A. R., Axelrod, H. L., Cohen, A. E., Abresch, E. C., Zuris, J., Yee, D., ... & Paddock, M. L. (2009). Crystal structure of Miner1: The redox-active 2Fe-2S protein causative in Wolfram Syndrome 2. Journal of molecular biology, 392(1), 143-153. PMID: 19580816 PMC2739586 DOI: 10.1016/j.jmb.2009.06.079
- ↑ Cattaneo, M., La Sala, L., Rondinelli, M., Errichiello, E., Zuffardi, O., Puca, A. A., ... & Ceriello, A. (2017). A donor splice site mutation in CISD2 generates multiple truncated, non-functional isoforms in Wolfram syndrome type 2 patients. BMC Medical Genetics, 18(1), 1-8. PMID: 29237418 PMC5729406 DOI: 10.1186/s12881-017-0508-2
- ↑ Callens, M., Loncke, J., & Bultynck, G. (2022). Dysregulated Ca2+ Homeostasis as a Central Theme in Neurodegeneration: Lessons from Alzheimer’s Disease and Wolfram Syndrome. Cells, 11(12), 1963. PMID: 35741091 PMC9221778 DOI: 10.3390/cells11121963
- ↑ Chen, Y. F., Wu, C. Y., Kirby, R., Kao, C. H., & Tsai, T. F. (2010). A role for the CISD2 gene in lifespan control and human disease. Annals of the New York Academy of Sciences, 1201(1), 58-64. https://doi.org/10.1111/j.1749-6632.2010.05619.x
- ↑ Amr, S., Heisey, C., Zhang, M., Xia, X. J., Shows, K. H., Ajlouni, K., ... & Shiang, R. (2007). A homozygous mutation in a novel zinc-finger protein, ERIS, is responsible for Wolfram syndrome 2. The American Journal of Human Genetics, 81(4), 673-683. https://doi.org/10.1086/520961
- ↑ Chen, Y. F., Kao, C. H., Chen, Y. T., Wang, C. H., Wu, C. Y., Tsai, C. Y., ... & Tsai, T. F. (2009). Cisd2 deficiency drives premature aging and causes mitochondria-mediated defects in mice. Genes & development, 23(10), 1183-1194. PMID: 19451219 PMC:2685531 DOI: 10.1101/gad.1779509
- ↑ 19.0 19.1 Teng, Y. C., Wang, J. Y., Chi, Y. H., & Tsai, T. F. (2020). Exercise and the Cisd2 Prolongevity Gene: Two Promising Strategies to Delay the Aging of Skeletal Muscle. Int. J. Mol. Sci, 21, 9059. PMC:7731423 Doi:10.3390/ijms21239059
- ↑ Yeh, C. H., Shen, Z. Q., Hsiung, S. Y., Wu, P. C., Teng, Y. C., Chou, Y. J., ... & Tsai, T. F. (2019). Cisd2 is essential to delaying cardiac aging and to maintaining heart functions. PLoS biology, 17(10), e3000508. PMID: 31593566 PMC:6799937 DOI:10.1371/journal.pbio.3000508
- ↑ 21.0 21.1 21.2 Wu, C. Y., Chen, Y. F., Wang, C. H., Kao, C. H., Zhuang, H. W., Chen, C. C., ... & Tsai, T. F. (2012). A persistent level of Cisd2 extends healthy lifespan and delays aging in mice. Human molecular genetics, 21(18), 3956-3968. PMID: 22661501 DOI:10.1093/hmg/dds210
- ↑ Karmi, O., Rowland, L., King, S. D., Manrique‐Acevedo, C., Cabantchik, I. Z., Nechushtai, R., & Mittler, R. (2022). The [2Fe‐2S] protein CISD2 plays a key role in preventing iron accumulation in cardiomyocytes. FEBS letters, 596(6), 747-761. PMID: 34997963 DOI: 10.1002/1873-3468.14277
- ↑ Yeh, C. H., Chou, Y. J., Chu, T. K., & Tsai, T. F. (2021). Rejuvenating the aging heart by enhancing the expression of the cisd2 prolongevity gene. International journal of molecular sciences, 22(21), 11487. PMID: 34768917 PMC:8583758 DOI:10.3390/ijms222111487
- ↑ 24.0 24.1 24.2 Yeh, C. H., Shen, Z. Q., Lin, C. C., Lu, C. K., & Tsai, T. F. (2022). Rejuvenation: Turning Back Time by Enhancing CISD2. International Journal of Molecular Sciences, 23(22), 14014. https://doi.org/10.3390/ijms232214014
- ↑ 25.0 25.1 Yeh, C. H., Shen, Z. Q., Wang, T. W., Kao, C. H., Teng, Y. C., Yeh, T. K., ... & Tsai, T. F. (2022). Hesperetin promotes longevity and delays aging via activation of Cisd2 in naturally aged mice. Journal of biomedical science, 29(1), 1-21. PMID: 35871686 PMC9310407 DOI: 10.1186/s12929-022-00838-7
- ↑ Liu, P., Li, J., Liu, M., Zhang, M., Xue, Y., Zhang, Y., ... & Chu, L. (2021). Hesperetin modulates the Sirt1/Nrf2 signaling pathway in counteracting myocardial ischemia through suppression of oxidative stress, inflammation, and apoptosis. Biomedicine & Pharmacotherapy, 139, 111552. PMID: 33839495 DOI:10.1016/j.biopha.2021.111552
- ↑ Pandey, P., & Khan, F. (2021). A mechanistic review of the anticancer potential of hesperidin, a natural flavonoid from citrus fruits. Nutrition Research, 92, 21-31. PMID: 34273640 DOI:10.1016/j.nutres.2021.05.011
- ↑ Yap, K. M., Sekar, M., Wu, Y. S., Gan, S. H., Rani, N. N. I. M., Seow, L. J., ... & Lum, P. T. (2021). Hesperidin and its aglycone hesperetin in breast cancer therapy: A review of recent developments and future prospects. Saudi Journal of Biological Sciences, 28(12), 6730-6747 PMID:34866972 PMC:PMC8626310 DOI: 10.1016/j.sjbs.2021.07.046.
- ↑ Yang, H., Wang, Y., Xu, S., Ren, J., Tang, L., Gong, J., ... & Su, D. (2022). Hesperetin, a promising treatment option for diabetes and related complications: A literature review. Journal of Agricultural and Food Chemistry, 70(28), 8582-8592. PMID:35801973 DOI:10.1021/acs.jafc.2c03257
- ↑ 30.0 30.1 30.2 Wdowiak, K., Walkowiak, J., Pietrzak, R., Bazan-Woźniak, A., & Cielecka-Piontek, J. (2022). Bioavailability of Hesperidin and Its Aglycone Hesperetin—Compounds Found in Citrus Fruits as a Parameter Conditioning the Pro-Health Potential (Neuroprotective and Antidiabetic Activity)—Mini-Review. Nutrients, 14(13), 2647. PMID: 35807828 PMC9268531 DOI: 10.3390/nu14132647
- ↑ Ortiz, A. D. C., Fideles, S. O. M., Reis, C. H. B., Bellini, M. Z., Pereira, E. D. S. B. M., Pilon, J. P. G., ... & Buchaim, R. L. (2022). Therapeutic Effects of Citrus Flavonoids Neohesperidin, Hesperidin and Its Aglycone, Hesperetin on Bone Health. Biomolecules, 12(5), 626. PMID:35625554 PMC:9138288 DOI: 10.3390/biom12050626
- ↑ Man, M. Q., Yang, B., & Elias, P. M. (2019). Benefits of hesperidin for cutaneous functions. Evidence-Based Complementary and Alternative Medicine, 2019. PMID: 31061668 PMC:6466919 DOI: 10.1155/2019/2676307
- ↑ Evans, J. A., Mendonca, P., & Soliman, K. F. (2022). Neuroprotective Effects and Therapeutic Potential of the Citrus Flavonoid Hesperetin in Neurodegenerative Diseases. Nutrients, 14(11), 2228. PMID:35684025 PMC:9183194 DOI: 10.3390/nu14112228
- ↑ ur Rehman, M. F., Batool, A. I., Qadir, R., & Aslam, M. (2021). Hesperidin and naringenin. In A centum of valuable plant bioactives (pp. 403-444). Academic Press. https://doi.org/10.1016/B978-0-12-822923-1.00027-3
- ↑ Hesperidin in Peppermint, dried
- ↑ Erlund, I. (2004). Review of the flavonoids quercetin, hesperetin, and naringenin. Dietary sources, bioactivities, bioavailability, and epidemiology. Nutrition research, 24(10), 851-874. https://doi.org/10.1016/j.nutres.2004.07.005
- ↑ Sohel, M., Sultana, H., Sultana, T., Al Amin, M., Aktar, S., Ali, M. C., ... & Dash, R. (2022). Chemotherapeutic potential of hesperetin for cancer treatment, with mechanistic insights: a comprehensive review. Heliyon, e08815.PMID: 35128104 PMC8810372 DOI: 10.1016/j.heliyon.2022.e08815
- ↑ Wdowiak, K., Rosiak, N., Tykarska, E., Żarowski, M., Płazińska, A., Płaziński, W., & Cielecka-Piontek, J. (2022). Amorphous Inclusion Complexes: Molecular Interactions of Hesperidin and Hesperetin with HP-Β-CD and Their Biological Effects. International journal of molecular sciences, 23(7), 4000. PMID: 35409360 PMC:9000012 DOI: 10.3390/ijms23074000
- ↑ Rosiak, N., Wdowiak, K., Tykarska, E., & Cielecka-Piontek, J. (2022). Amorphous Solid Dispersion of Hesperidin with Polymer Excipients for Enhanced Apparent Solubility as a More Effective Approach to the Treatment of Civilization Diseases. International Journal of Molecular Sciences, 23(23), 15198. https://doi.org/10.3390/ijms232315198
- ↑ Lin, C. C., Chiang, T. H., Chen, W. J., Sun, Y. Y., Lee, Y. H., & Lin, M. S. (2015). CISD2 serves a novel role as a suppressor of nitric oxide signalling and curcumin increases CISD2 expression in spinal cord injuries. Injury, 46(12), 2341-2350. PMID: 26387034 DOI:10.1016/j.injury.2015.07.040
- ↑ Lin, C. C., Chiang, T. H., Sun, Y. Y., & Lin, M. S. (2019). Protective effects of CISD2 and influence of curcumin on CISD2 expression in aged animals and inflammatory cell model. Nutrients, 11(3), 700. PMID: 30934593 PMC6470567 DOI: 10.3390/nu11030700