Aging and longevity
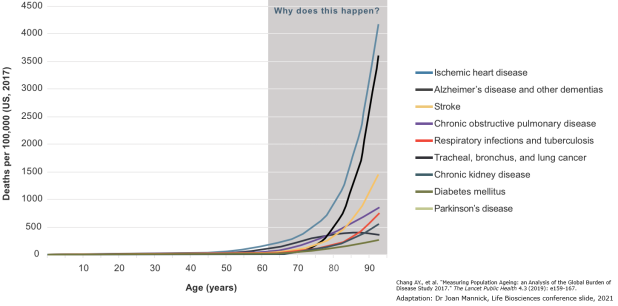
People have yearned for the fountain of youth all throughout history. However, until recently, there has not been a concerted research effort to address biological aging as a cause of disease. In the modern era, humanity supports research towards the cure of age-related diseases (e.g. Alzheimer’s, heart disease, and most cancers) – a desire present at every level of human organization that spans across individuals, communities, and society. This reflects a core human desire to be healthy. Yet, recognizing that humanity should strive towards a cure for biological aging is a sentiment that does not attract such widespread popularity.
Lack of societal support stems from two fundamental misconceptions – that ‘aging’ merely represents the natural chronological passage of time, and that aging is an entirely separate category from age-related disease.[1] In contrast, the biology of aging research field (biogerontology) views the mechanisms of aging as the root cause of most of society’s most prevalent, costly, and debilitating diseases.[2][3][4]
In 2018, for the first time in human history, the number of people aged over 64 became greater than those aged under 5.[5] This disparity is projected to widen with a rapidly aging population.[5] The clear implications for a substantial increase in age-related disease burden has led to urgent calls from scientists for global society to support aging biology research to increase healthy life expectancy.[4][6]
What is aging?
Aging is an imprecise term with multiple definitions that depend on context. It may describe a time-dependent process in a physical, biological or sociological sense, and apply to the level of molecules, organisms, or inanimate objects.[7][8] Even when narrowly considered in a biological context, an exact consensus definition is difficult among researchers for such a complex phenomenon.[9]
There is now a rapidly growing base of biogerontology researchers that study the biology of aging. This discipline of biomedical science is specifically interested in how the molecular mechanisms of aging are fundamental to the development of age-related diseases, and how these mechanisms unite seemingly unrelated diseases of aging.[10]
Medically speaking, aging may be thought of as the progressive physiological decline in the body’s resilience and ability to overcome stressors.[11][12] Aging-related decline in physical and mental capacity leads to permanent loss of function, independence, and dignity.[13][14] This occurs due to the accumulation of multiple age-related diseases (ARDs), leading to an extended period of suffering from mid to late life.[15] Eventually, people die of a specific ARD, but many will suffer from multiple such diseases before death. Indeed, it is a common misconception that the elderly die of 'old age', although this term is useful when a specific cause of death is elusive.
Over the last few decades, biomedical scientists have identified several Hallmarks of Aging[16] that represent fundamental biological mechanisms of aging. These hallmarks form a basis for how aging might be targeted to slow, stop, or even reverse multiple age-related diseases. There are various theories for what is or what causes biological aging[17][18] - though there remains no consensus - and some argue that aging is not a unitary phenomenon.[18][19] However, it is now widely accepted among biogerontology researchers that various aging processes, such as the Hallmarks of Aging, may be considered for medical intervention. This change in thinking is reflected by the dramatic increase in biotech companies and human clinical trials targeting aging.[20]
The current approach to medicine is generally focused on increasing lifespan by attempting to cure single diseases. In contrast, therapies that target biological aging are hypothesized to uniquely have the ability to significantly increase healthy lifespan. This is because the latter approach addresses what appears to be the fundamental biological mechanisms that unite ostensibly unrelated age-related diseases.[21] This distinction is supported by decades of experimental evidence in animals, where the biology of aging is manipulated. It may also be inferred theoretically: If the incidence of all age-related diseases increases in an exponential relationship from progressive systemic decline with age, then eliminating any single age-related disease would achieve little, as it would merely allow one to develop another age-related disease soon after.
Animal studies reveal that aging is malleable
Numerous experimental breakthroughs over the last few decades have shown that, contrary to prevailing thought, biological aging is medically treatable in animals. Slowing or even hastening of aging in animals can be achieved via manipulation of diet, environment, genetics, epigenetics, or with drugs, with experiments ranging from roundworms to mice and even non-human primates.[22][23][24][25][26] Among the hundreds of aging laboratories across the world, aging is routinely manipulated by biogerontology researchers. This is a global endeavor attempting to understand what aging is, and to determine how this knowledge may be used to develop medicines for all of humanity.
Senolytics
An example of the striking differences in health that are possible with therapies that target aging biology can be seen in animal experiments of senolytics, first conducted by researchers at the Mayo Clinic.
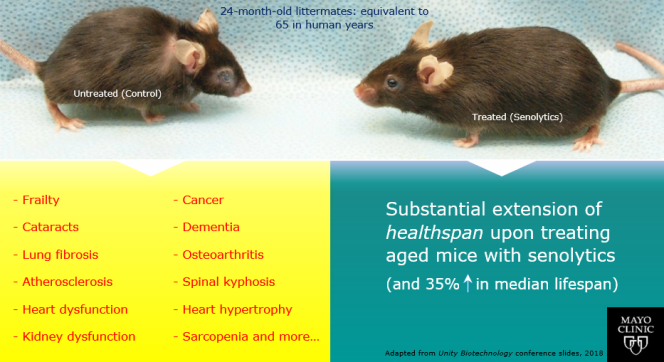
Senescent cells are known to accumulate with aging throughout the body, and drive multiple age-related diseases. Experiments done in mice show that removing these cells with drugs that clear these cells - senolytics - restores health. This is because senolytics can slow and even partially reverse aging, which leads to increased healthspan from addressing multiple age-related diseases. As a side effect of the old mice being rejuvenated, studies have observed increases in median lifespan of up to 35%.
Epigenetic reprogramming
Recent experimental breakthroughs have provided early evidence that aspects of aging can be reversed,[27][28] challenging old assumptions that aging is simply a result of time passing.
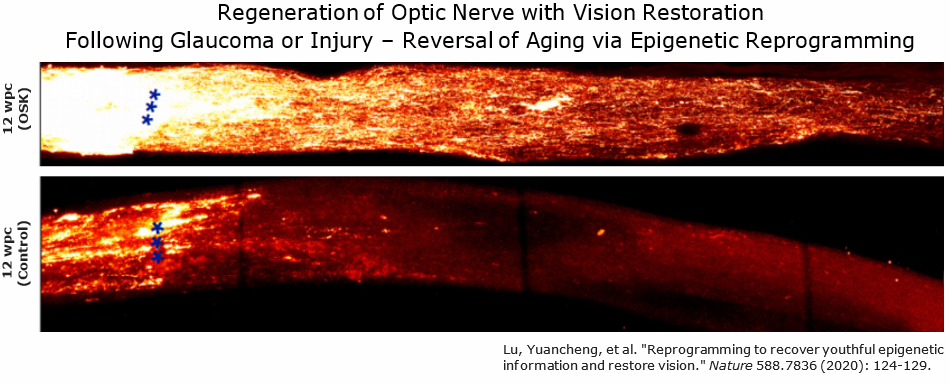
Epigenetic reprogramming is based on work that earnt Shinya Yamanaka the 2012 Nobel Prize in Medicine. Yamanaka showed that it was possible to reprogram adult body cells back into biologically immortal pluripotent stem cells that are capable of turning into any cell of the body.[29][30] This was achieved by activating only four transcription factors - the Yamanaka factors - OCT4, SOX2, KLF4, and MYC (or OSKM).[29][30]
Scientists in the aging biology field have since expanded upon this work to show that a partial version of this epigenetic reprogramming technique may reverse multiple aspects of aging.[27] Work from Juan Carlos Izpisua Belmonte's lab showed that it is possible to modify the reprogramming technique and achieve 'youthful' rejuvenation, without resetting a cell with a defined identity into a stem cell. This research was published in 2016, when the Belmonte lab first showed that temporarily expressing the Yamanaka factors in a controlled manner could rejuvenate multiple aged organs and extend healthy lifespan in mice with accelerated aging.[31][32]
Using epigenetic reprogramming in naturally aged mice has more recently been shown to improve memory/cognition, promote muscle regeneration, and restore vision.[33][34][28] It has been envisioned by some scientists as a therapy that could be used periodically, perhaps every few decades, to continually reverse aging in humans. However, evidence for a truly comprehensive reversal of aging via partial reprogramming in mice, let alone in humans, is lacking. Whether reprogramming based strategies will extend healthy lifespan in normally aged mice is not currently well studied.
Dietary restriction mimetics and mTOR inhibitors
Dietary restriction (DR) has consistently been shown to extend healthy lifespan in animals ranging from worms, to mice, and to primates.[35][36] In animals, DR leads to significant improvement to healthy lifespan; however, the proportional lifespan benefit generally declines with increasing species size/complexity.[37][38] Like many therapies that target aging, the effect of DR is not consistent across different studies for various reasons.[39][40] This is often due to genetic factors, such as several studies showing that while certain strains of mice subjected to DR live longer, other strains show no benefit or even lifespan detriment.[39][40] Other reasons may be related to environmental differences, or DR extent or diet composition in DR diets used in these experiments.
Dysregulation of nutrient-sensing is regarded as a hallmark of aging, playing a key role in the aging process.[16] Relevant metabolic pathways such as IGF-1, mTOR and AMPK, are influenced by DR in extending healthy lifespan in animals.[41]
Given the difficulty of implementing DR as medicine for humans, due to difficulty with patient adherence, drugs that mimic the effects of dietary restriction are being developed.[38] Similarly, there is a significant and ongoing research effort that aims to determine whether intermittent fasting or time restricted eating may capture some of the potential health benefits of DR, while being easier for patients to adhere to.[37] There is generally a lack of evidence in human clinical trials in justifying the use of these lifestyle interventions for slowing aging in healthy adults.[42]
Metformin
Metformin extends healthy lifespan in worms, mice and rats.[43][44] Metformin is one of the cheapest and most commonly prescribed medications in the world, and has been linked to lower incidence of cancer, heart disease, dementia, and mortality in patients with type 2 diabetes (T2DM).[45]
The Targeting Aging with Metformin (TAME) trial is investigating a drug with over 6 decades of human use, currently the first-line therapy for the age-related disease T2DM. One study suggested that despite the life-shortening effects of diabetes, T2DM patients on metformin, but not other medications, outlive those who are not diabetic.[46] As metformin has also shown healthspan and lifespan extension effects in naturally aging worms and mice, some researchers believe that it may slow aging.[45] However, the data in humans is inconclusive without formal testing, such as in a randomized clinical trial (RCT).
The Targeting Aging with Metformin (TAME) trial is a US, nation-wide, six-year RCT of over 3000 individuals aged 65 to 79.[45] The goal is to investigate whether metformin will slow aging in humans, as it has been shown to extend healthspan in some animals. This will be measured by whether metformin can prevent the onset of multiple age-related diseases such as heart disease, cancer, and dementia.
A key defining aspect in qualifying whether a drug targets aging (a gerotherapeutic or geroprotective drug), is the ability to delay or reverse the onset of multiple age-related diseases in tandem, to increase healthy lifespan.[47] The aging field continues to debate whether a geroprotector necessarily needs to increase healthspan, or both healthspan and lifespan.[48] Yet, what is clear now is that aging has a biology of critical relevance to the diseases associated with aging. This, along with multiple other lines of evidence across biology and epidemiology, suggest that the accumulation of multiple age-related diseases with time are not simply disparate processes or chance events that bear no relationship with one another. These diseases of older age, may in fact, be driven by an underlying aging process.[2][3][4]
Rapamycin
Rapamycin has been shown to extend healthy lifespan in worms, yeast, flies, and mice.[49] Some physicians and scientists have thus suggested that rapamycin may slow down aging.[50][51] It is currently an approved drug used to prevent the rejection of organ transplants by the immune system. Rapamycin is a natural antifungal produced by soil bacteria of Eastern Island, named after its native island, Rapa Nui.[52]
Scientists who study the biology of aging believe that, due to evidence of rapamycin slowing aging in animals, it could be used to prevent the onset of all age-related diseases in humans – to become one of the first identified “anti-aging drugs”.[50][53]
Among the hundreds of interventions studied within geroscience research, rapamycin is unique in its highly consistent and reproducible effect on healthy lifespan extension in mice.[54] It is also the drug that exhibits the largest lifespan effect extension in multiple strains of mice, both when dosed in early life and at old age.[55][56] Rapamycin is being repurposed for humans as an anti-aging drug, such as in the PEARL clinical study, which is expected to provide preliminary results in 2023.[57]
The link between lifespan and aging
- Lifespan can range from mere days in mayflies, to several years in dogs, and up to centuries in bowhead whales[58]
- Studying aging in animals has led to an understanding that aging is biological and not a mere consequence of time[2]
- Research in a range of different animals show that there exists extreme variance in rates of aging, within and across species[2]
- Humans have been observed to exhibit substantial variance in rates of aging, both in terms of accelerated and decelerated aging[59]
- Centenarians (those living to age 100 or more) live longer due to a delayed onset of all age-related diseases, influenced primarily by genetics[60]
- Not only do centenarians live longer, but the period of suffering in late life from age-related diseases is also far shorter than non-centenarians - known as the compression of morbidity[61]
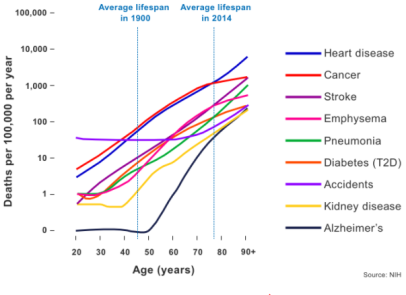
The totality of age-related diseases is prohibitively long to list in text, but includes Alzheimer’s, cardiovascular disease, most cancers, stroke, osteoarthritis, macular degeneration, and COVID-19.
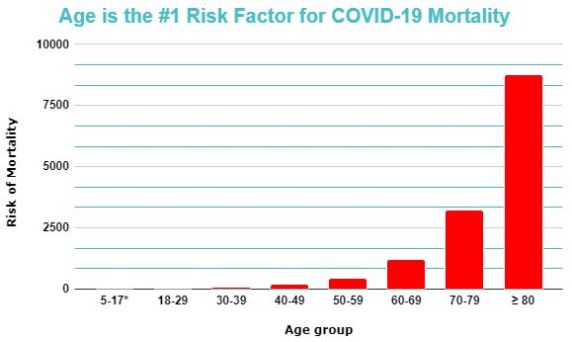
Age-related diseases can be identified by a characteristic exponential increase in incidence with age, following the Gompertz-Makeham law of mortality. This law essentially describes how all-cause mortality rate doubles every 8 years, which is a relationship common to age-related diseases alone.
COVID-19 defined as a disease of aging
As detailed in the paper "COVID‐19 is an emergent disease of aging",[62] COVID-19 meets several criteria for definition as an age-related disease:[63][64][65][66][67]
1) The doubling time for COVID-19 mortality approaches that of the doubling time of 8 years for all-cause mortality (characteristic exponential increase shared among major age-related chronic diseases);
2) Greater mortality rate in men, consistent with known sex differences in rates of aging;
3) Greater mortality rate for those with comorbidities (age-related diseases), consistent with accelerated biological aging; and,
4) Age dwarfs all other putative risk factors for mortality by several orders of magnitude, exhibiting several thousand fold increase in risk across the lifespan
Risk factors are used medicine to assess individual and population risks for certain diseases, in order to guide diagnosis and clinical management. An example of a risk factor is smoking status, which increases the risk of lung cancer by approximately 7 fold.[68]
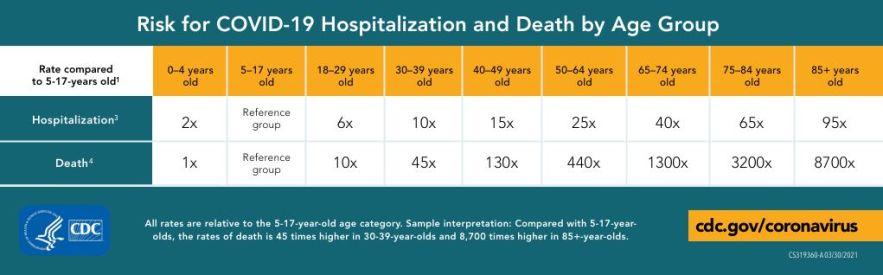
As compared to a reference group aged 5-14, the risk of death for COVID-19 is 8700 fold greater in those aged over 85, as per the US Center for Disease Control (CDC). Common independent risk factors identified in scientific discourse are comparatively inconsequential; for example, a history of chronic lung disease confers only a 2-fold risk of COVID-19 mortality, despite the reputation as a respiratory disease.[69] The epidemiological data also makes clear that 'healthy' older adults with one or no comorbidities are still at substantially greater risk of severe disease than in the young. More importantly, those with accelerated biological aging with multiple comorbidities of age are at even greater risk.[70] This not only highlights how age is the ultimate risk factor for mortality, but also emphasizes the importance of biological age.
COVID-19 results in systemic involvement with neurologic, kidney, liver, heart, endocrine, and gut complications.[71] Therefore, testing of geroprotectors that target multiple aged and vulnerable organ systems in clinical trials is hypothesized to be desirable.[65][66] What is important about SARS-CoV-2 is that not only does the virus have many consequences across the entire body, but it also disproportionately affects those with higher biological age. Therefore, an emphasis on the specific organ system of respiration (or the virus itself), instead of on the aging host, may be regarded by biogerontology researchers as a short-sighted therapeutic strategy in the long-term.[65] This is especially important for preventing future pandemics, as well as to address multiple other age-related diseases of an aging population that is burdening global healthcare systems.
Traditional risk factors and heart disease
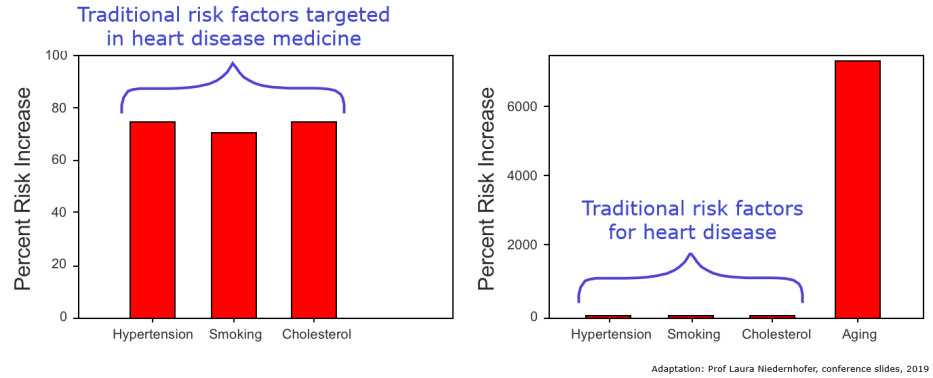
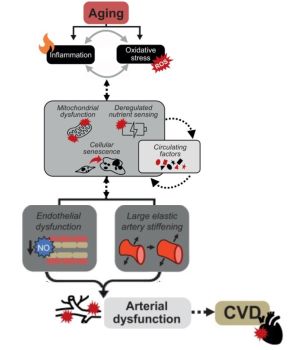
Hypertension, smoking, and cholesterol are examples of well-known heart disease risk factors that have been used by scientists/physicians to guide the research and development of effective therapies. This strategy originated from the Framingham Heart Study – an initiative spanning decades that observed thousands of people to understand what genetic and environmental factors influence heart disease.[73]The resulting risk factor paradigm has since been adopted across all domains of disease in biomedical research, and is core to the practice of clinical medicine Coordinated research efforts have helped identify genetic and environmental factors important to heart disease risk. Identifying whether risk factors are modifiable enables the development of effective therapeutics, such as for the prevention of cardiovascular diseases. For example, identifying high blood pressure and cholesterol as modifiable risk factors has since led to anti-hypertensive and cholesterol medications being among the most commonly prescribed drugs across the world.[74] Aging is widely accepted among physicians and biomedical scientists as the greatest risk factor for almost all the major diseases in developed countries.[75] However, aging is also traditionally viewed as a risk factor that cannot be modified. Unmodifiable risk factors are regarded as unimportant for the development of interventions. The prevailing assumption that aging is an unmodifiable risk factor is now being challenged by biogerontology researchers.[76]
The overall exponential relationship between increasing age and mortality is consistent for various age-related diseases, regardless of whether a disease is acute or chronic. This reflects the continually increasing vulnerability and decreasing resilience of the human body with age.
Why is population aging a problem?
Often described as the ‘climate change of healthcare’, an aging population comes with challenges due to declines in productivity, loss of independence, and increasingly unsustainable healthcare costs.
In 2018, human civilisation reached an unprecedented point in history. People aged over 65 now outnumber those aged under 5, and this disparity is projected only to widen across the world as the global population ages and fertility rates continue to decline.[77][78]
The aging population is a major contributor to increasing global healthcare costs, which have risen steadily since the 1900s and are projected only to grow further.[79] These costs are increasingly unsustainable, and demographic change is also expected to place a greater burden on society due to increased dependency and reduced productivity.
The COVID-19 pandemic is an example of what a single age-related disease can do to an aging society. US CDC statistics show that 97.11% of mortality occurs in those aged over 45, and the exponential increase in mortality risk with age is essentially identical to all other leading causes of death and suffering, such as for cardiovascular diseases and cancer.[62] The pandemic has highlighted the vulnerability of our aging population, and foreshadows an impending healthcare crisis of an aging world - the climate change of healthcare.
Aging is not just a problem for the ‘elderly’, as various aspects of aging begin well before middle-age. Many people suffer from accelerated aging and develop multiple age-related diseases prematurely, such as with depression, stress, poverty, HIV/AIDs, diabetes, smoking, Down Syndrome, accelerated aging syndromes (e.g. progerias) and in childhood cancer survivors.[80][81][82][83][84][85][86][87][88][89][90]
As a result of the potential for rejuvenation of multiple tissues and organs which has been seen in aging biology research, the applications of such research to human health are not merely limited to age-related diseases. In addition to the above-mentioned examples of accelerated aging, there are also significant implications for orphan or rare diseases.
Economic implications of targeting aging
In 2019, the Bank of America projected the longevity industry to reach $600 billion by 2025.[91] This is nearly a six-fold increase over 6 years, reflecting the burgeoning interest in aging biology research.
A recent paper published by Professors at Harvard Medical School, Oxford University, and London Business School, estimated that a drug that slows aging by merely one year could add $US 38 trillion to the US economy.[92] This was justified by estimating how slowed aging can result in both improved health and longevity. The resultant increases in independence and productivity is subject to a virtuous cycle of further gains.[92] This analysis highlights the profound difference between the current approach of targeting single diseases, versus targeting aging.
Healthspan versus lifespan
The concept of healthspan refers to the period of life spent free from disease
In the 21st Century, population healthspan contrasts significantly with lifespan. Although the latter has steadily increased over the last two centuries, the evidence strongly suggests that healthspan has not kept up with lifespan.[21]
Advances in various public health measures, substantial reductions in childhood mortality, and effective treatments for several leading causes of death such as cardiovascular diseases have led to increased human life expectancy.[79][21] However, many human populations now spend a greater proportion of life in ill health.[79][21][93] Reduced mortality from specific diseases may allow humans to survive into older age, but the quantity of life gained is minimal due to diminishing returns from competing risks of mortality.[94][95] More importantly, this is permissive for increased periods of suffering from typically non-fatal conditions such as osteoarthritis or cognitive decline.[21]
Single disease medicine
A major problem in the current healthcare strategy for an aging population lies in the traditional medical paradigm of 'one disease at a time' medicine.[96]
The infectious disease model of medicine, which originates from germ theory, accounts for historic human public health achievements of the 20th Century. These include substantial reductions in mortality from communicable diseases, such as smallpox, measles, and HIV/AIDS.[97][98] This paradigm relies on disease classifications characterized by sets of symptoms and signs, which are then treated with intervention(s) targeting the given disease. Treating diseases separately as they come, one by one, defines the current strategy to medicine.
The current approach to medicine is often described by proponents of preventive medicine as 'sick care' rather than healthcare. The overall approach is to wait until people get sick with various chronic diseases, and then attempt to treat them. In healthcare, there is little emphasis on keeping aging people 'healthy', at least as compared to what is commonly regarded as youthful health.[96] From the perspective of geroscience, the physiological changes with aging that precede disease should be considered within the domain of medicine. While originally used for acute diseases such as the Flu, this paradigm has since permeated into chronic diseases that persist in the long-term.
Additionally, it can be inferred from the current approach to medicine of the belief that ideal medical outcomes come from an approach that subcategorizes into organ or physiologic systems. This is reflected in increasing subspecialization. For example, for type 2 diabetes, patients typically begin with a general practitioner; further disease progression may then involve physicians specializing in endocrinology, or even further with diabetology. At the level of single diseases, such specialization can lead to better outcomes. But at the level of population health and in the context of aging, this fails to recognize the complexities of multimorbidity, the age-related development of multiple chronic diseases.
The Taeuber Paradox
Assuming that the goal of medicine should be to maximize both quality and length of life, the single disease medicine paradigm is theoretically flawed. Even if a cure for a specific age-related disease were possible, the exponential increase in risk of death from other competing diseases continues unabated.[96]This flaw can be further understood via the Taeuber Paradox:
Completely curing heart disease or cancer, which are among the leading causes of deaths globally, is estimated to each add only ~2.5 years to life expectancy.[94][95] Eliminating Alzheimer's disease as a cause of death would add only 2 months to life expectancy.[99][100]
Additionally, such cures would have minimal or even detrimental effects on healthspan. For example, it is well known that the stress induced by cancer therapies accelerates aging.[101] Childhood cancer survivors develop heart disease, Alzheimer’s, frailty and other age-related diseases years or even decades earlier.[89]
Aiming to cure diseases one at a time, instead of addressing aging itself, results in diminishing returns due to competing risks of morbidity and mortality. Delaying heart disease with cholesterol medications does not address incipient frailty, preventing fatality from kidney disease may result in eventual Alzheimer’s. Indeed, the modern rise in Alzheimer's prevalence may have come about due to successes in delaying cardiovascular diseases, which are major causes of death.
The geroscience hypothesis
‘Geroscience’ represents the maturation of the research field of aging biology, transitioning from a research mainly concerned with animal aging, to one that is now concerned with human aging. The fundamental link between aging and age-related disease has been identified as the geroscience hypothesis.[14] This hypothesis posits that there are foundational biological mechanisms or 'pillars' of aging shared between apparently unrelated age-related diseases, which may be targeted for intervention to address multiple diseases in tandem.[14][16]
In other words, if aging is the primary cause of the various diseases that accumulate with age, then targeting this biology will treat or prevent multiple diseases. This affords a far greater potential to extend healthy lifespan than single-disease approaches. The geroscience hypothesis could therefore, within the context of an aging population, bring about a paradigm shift in the approach to medicine.
"There has been a shift in how we have considered aging, from something that we needed to account for and eliminate by statistical adjustment to a causal factor in disease...understanding aging provides the strongest chance to prevent chronic diseases and expand healthspan"[11]
Luigi Ferrucci, MD/PhD, Scientific Director of the National Institute on Aging (NIA, of the US National Institutes of Health)
One of the key aspects that distinguishes a geroprotector from medicine developed in the past is that the former may address aging as the primary cause of age-related diseases. The term 'cause' is not typically used rigorously in the biomedical sciences. Strictly speaking, a factor that causes a disease implies it is the (major) reason for developing a disease; however, the risk of developing a majority of diseases depends on many factors that each play small roles. Acceleration and deceleration of biological aging influences the development of various diseases that share age as the major risk factor (but are otherwise apparently unrelated). Based on experimental evidence in animals, as well as examples of slowed aging such as in centenarians, there is a case to be made that aging is a primary cause of age-related diseases.[2][60]
Is aging a disease?
Aging is often regarded as a natural and universal fact of life. In medicine, physicians are tasked with the need to classify whether the patient in front of them has a disease or not. This is a necessary part of the current approach of disease-centric medicine, because a binary decision is made to determine whether treatment is necessary. Under this paradigm, age-related changes not regarded as outright disease may be deemed normal, and so further management is not necessary. Such change with age may be related to function, such as declines in grip strength or exercise capacity; or physiological, such as increased low-grade systemic inflammation.[102][103] What may be regarded as normal or healthy in clinical practice is also typically compared to chronological age, i.e. being healthy for one's age. This can neglect the reality that a healthy 60 year old is functionally impaired compared to the average 20 year old.[104]
Lack of consensus on what defines aging
However, some level of collective confusion in meaning is prevalent among the public, physicians, and scientists. This is predominantly due to the imprecision of the word 'aging'. Even among aging biology researchers there is a lack of consensus as to what aging refers to.[9] This remains a significant problem among scientists, and is regarded by some as a barrier to advancing the field into mainstream public consciousness. Yet, there is now consensus among geroscience researchers (the subfield of aging biology concerned with medicine) that aging mechanisms can be targeted to increase healthspan.
Whether aging is a disease or not is regarded by some researchers as a mere semantic problem. This is as it does not directly interfere with the goals of geroscience. Researchers in the field believe that targeting aging biology will be integral to addressing the ongoing and growing public health crisis of an aging population.
Inconsistencies in criteria for defining a disease
There are various historical examples of prevalent diseases that were once accepted as normal, but have since become thought of as diseases.[105] Criteria for disease definitions are often highly inconsistent or debated, and influenced significantly by evolving sociocultural factors.[105][106] For example, before effective treatments for tuberculosis were created, it was commonly regarded as natural and to be accepted. Tuberculosis was actively glorified and romanticized.[107][108]
Others have argued that if an ailment affects everyone, it should not be classified as a disease, which is a common talking point against calling aging a disease.[109] Atherosclerosis, the hardening of arteries, is near ubiquitous with aging, yet has disease status.[110] Despite prevalence approaching 100% with age, blood pressure and cholesterol medications are widely prescribed to prevent atherosclerosis.[110] In a paper published by the inventor of statins, Akira Endo comments: "Serious research on the role of cholesterol in human atherosclerosis did not really get underway until the 1940s, due to a prevailing view that the disease was a simple consequence of aging and could not be prevented." A more recent example is sarcopenia, a term referring to the age-related, progressive loss of muscle function. In 2016, sarcopenia was added to the World Health Organization's ICD-10 list of diseases.[111]
Disease definitions in medicine are continually changing, and often depending on whether we have treatments for a given disease or not. This has implications for regulation, such as in considering the scope of the Federal Drug Administration (FDA) in the US. Additionally, classification as a disease spurs further research into developing diagnostics, and also for pharmaceutical companies to develop treatments.[111]
There are growing numbers of aging researchers calling on governments to classify aging as a disease at a regulatory level, at least in the biological sense of the word as it relates to age-related disease.[112][113][114] Others contend that aging cannot be considered a disease, because aging affects everyone, and that aging is a natural part of life.[109][115] Another line of thought is that aging is neither a disease or not a disease. Whether it is a disease or not, if the biology of aging causatively influences health and vulnerability to disease, then there is moral imperative to develop medicines that alleviate such suffering.[116][117]
References
- ↑ Sierra, F. (2016). The emergence of geroscience as an interdisciplinary approach to the enhancement of health span and life span. Cold Spring Harbor perspectives in medicine, 6(4), a025163.
- ↑ 2.0 2.1 2.2 2.3 2.4 Campisi, J., Kapahi, P., Lithgow, G. J., Melov, S., Newman, J. C., & Verdin, E. (2019). From discoveries in ageing research to therapeutics for healthy ageing. Nature, 571(7764), 183-192.
- ↑ 3.0 3.1 Partridge, L., Fuentealba, M., & Kennedy, B. K. (2020). The quest to slow ageing through drug discovery. Nature Reviews Drug Discovery, 19(8), 513-532.
- ↑ 4.0 4.1 4.2 Longo, V. D., Antebi, A., Bartke, A., Barzilai, N., Brown‐Borg, H. M., Caruso, C., ... & Fontana, L. (2015). Interventions to slow aging in humans: are we ready?. Aging cell, 14(4), 497-510.
- ↑ 5.0 5.1 Ritchie, H., & Roser, M. (2019). Age structure. Our World in Data.
- ↑ Rae, M. J., Butler, R. N., Campisi, J., De Grey, A. D., Finch, C. E., Gough, M., ... & Logan, B. J. (2010). The demographic and biomedical case for late-life interventions in aging. Science translational medicine, 2(40), 40cm21-40cm21.
- ↑ Hayflick, L. (2002). Anarchy in Gerontological Terminology. Handbook of the Biology of Aging, edited by Edward J. Masoro and Steven N. Austad. Gerontologist, 42(3), 416-420.
- ↑ Hayflick, L. (2021). The greatest risk factor for the leading cause of death is ignored. Biogerontology, 22(1), 133-141.
- ↑ 9.0 9.1 Cohen, A. A., Kennedy, B. K., Anglas, U., Bronikowski, A. M., Deelen, J., Dufour, F., ... & Fülöp, T. (2020). Lack of consensus on an aging biology paradigm? A global survey reveals an agreement to disagree, and the need for an interdisciplinary framework. Mechanisms of ageing and development, 191, 111316.
- ↑ Franceschi, C., Garagnani, P., Morsiani, C., Conte, M., Santoro, A., Grignolio, A., ... & Salvioli, S. (2018). The continuum of aging and age-related diseases: common mechanisms but different rates. Frontiers in medicine, 5, 61.
- ↑ 11.0 11.1 Thuault, S. (2021). Reflections on aging research from within the National Institute on Aging. Nature Aging, 1(1), 14-18.
- ↑ Fabbri E, Zoli M, Gonzalez-Freire M, Salive ME, Studenski SA, Ferrucci L. Aging and multimorbidity: new tasks, priorities, and frontiers for integrated gerontological and clinical research. Journal of the American Medical Directors Association. 2015 Aug 1;16(8):640-7.
- ↑ de Grey, A.D. (2013). The desperate need for a biomedically useful definition of “aging”. Rejuvenation Research,16:89–90.
- ↑ 14.0 14.1 14.2 Kennedy, B. K., Berger, S. L., Brunet, A., Campisi, J., Cuervo, A. M., Epel, E. S., ... & Sierra, F. (2014). Geroscience: linking aging to chronic disease. Cell, 159(4), 709-713.
- ↑ Fabbri, E., Zoli, M., Gonzalez-Freire, M., Salive, M. E., Studenski, S. A., & Ferrucci, L. (2015). Aging and multimorbidity: new tasks, priorities, and frontiers for integrated gerontological and clinical research. Journal of the American Medical Directors Association, 16(8), 640-647.
- ↑ 16.0 16.1 16.2 López-Otín C, Blasco MA, Partridge L, Serrano M, Kroemer G. The hallmarks of aging. Cell. 2013 Jun 6;153(6):1194-217.
- ↑ Jin, K. (2010). Modern biological theories of aging. Aging and disease, 1(2), 72.
- ↑ 18.0 18.1 Cohen, A. A., Legault, V., & Fülöp, T. (2020). What if there’s no such thing as “aging”?. Mechanisms of Ageing and Development, 192, 111344.
- ↑ Peto, R., & Doll, R. (1997). There is no such thing as aging: Old age is associated with disease, but does not cause it.
- ↑ De Magalhães, J. P., Stevens, M., & Thornton, D. (2017). The business of anti-aging science. Trends in biotechnology, 35(11), 1062-1073.
- ↑ 21.0 21.1 21.2 21.3 21.4 Crimmins, E. M. (2015). Lifespan and healthspan: past, present, and promise. The Gerontologist, 55(6), 901-911.
- ↑ de Cabo, R., & Mattson, M. P. (2019). Effects of intermittent fasting on health, aging, and disease. New England Journal of Medicine, 381(26), 2541-2551.
- ↑ Keil, G., Cummings, E., & de Magalhaes, J. P. (2015). Being cool: how body temperature influences ageing and longevity. Biogerontology, 16(4), 383-397.
- ↑ Moskalev, A., Aliper, A., Smit-McBride, Z., Buzdin, A., & Zhavoronkov, A. (2014). Genetics and epigenetics of aging and longevity. Cell Cycle, 13(7), 1063-1077.
- ↑ Brunet, A., & Berger, S. L. (2014). Epigenetics of aging and aging-related disease. Journals of Gerontology Series A: Biomedical Sciences and Medical Sciences, 69(Suppl_1), S17-S20.
- ↑ Campisi, J., Kapahi, P., Lithgow, G. J., Melov, S., Newman, J. C., & Verdin, E. (2019). From discoveries in ageing research to therapeutics for healthy ageing. Nature, 571(7764), 183-192.
- ↑ 27.0 27.1 Ocampo, A., Reddy, P., Martinez-Redondo, P., Platero-Luengo, A., Hatanaka, F., Hishida, T., ... & Belmonte, J. C. I. (2016). In vivo amelioration of age-associated hallmarks by partial reprogramming. Cell, 167(7), 1719-1733.
- ↑ 28.0 28.1 Lu Y, Brommer B, Tian X, Krishnan A, Meer M, Wang C, Vera DL, Zeng Q, Yu D, Bonkowski MS, Yang JH. Reprogramming to recover youthful epigenetic information and restore vision. Nature. 2020 Dec;588(7836):124-9. https://www.nature.com/articles/s41586-020-2975-4
- ↑ 29.0 29.1 Takahashi, K., & Yamanaka, S. (2006). Induction of pluripotent stem cells from mouse embryonic and adult fibroblast cultures by defined factors. cell, 126(4), 663-676.
- ↑ 30.0 30.1 Takahashi, K., Tanabe, K., Ohnuki, M., Narita, M., Ichisaka, T., Tomoda, K., & Yamanaka, S. (2007). Induction of pluripotent stem cells from adult human fibroblasts by defined factors. cell, 131(5), 861-872.
- ↑ Ocampo, A., Reddy, P., Martinez-Redondo, P., Platero-Luengo, A., Hatanaka, F., Hishida, T., ... & Belmonte, J. C. I. (2016). In vivo amelioration of age-associated hallmarks by partial reprogramming. Cell, 167(7), 1719-1733.
- ↑ Rando, T. A., & Chang, H. Y. (2012). Aging, rejuvenation, and epigenetic reprogramming: resetting the aging clock. Cell, 148(1-2), 46-57.
- ↑ Wang, C., Ros, R. R., Martinez-Redondo, P., Ma, Z., Shi, L., Xue, Y., ... & Belmonte, J. C. I. (2021). In vivo partial reprogramming of myofibers promotes muscle regeneration by remodeling the stem cell niche. Nature Communications, 12(1), 1-15.
- ↑ Rodríguez-Matellán, A., Alcazar, N., Hernández, F., Serrano, M., & Ávila, J. (2020). In Vivo Reprogramming Ameliorates Aging Features in Dentate Gyrus Cells and Improves Memory in Mice. Stem cell reports, 15(5), 1056-1066.
- ↑ Fontana, L., Partridge, L., & Longo, V. D. (2010). Extending healthy life span—from yeast to humans. science, 328(5976), 321-326.
- ↑ Speakman, J. R., & Mitchell, S. E. (2011). Caloric restriction. Molecular aspects of medicine, 32(3), 159-221.
- ↑ 37.0 37.1 Katewa, S. D., & Kapahi, P. (2010). Dietary restriction and aging, 2009. Aging cell, 9(2), 105-112.
- ↑ 38.0 38.1 Selman, C. (2014). Dietary restriction and the pursuit of effective mimetics. Proceedings of the Nutrition Society, 73(2), 260-270.
- ↑ 39.0 39.1 Unnikrishnan, A., Matyi, S., Garrett, K., Ranjo‐Bishop, M., Allison, D. B., Ejima, K., ... & Richardson, A. (2021). Reevaluation of the effect of dietary restriction on different recombinant inbred lines of male and female mice. Aging Cell, e13500.
- ↑ 40.0 40.1 Liao, C. Y., Rikke, B. A., Johnson, T. E., Diaz, V., & Nelson, J. F. (2010). Genetic variation in the murine lifespan response to dietary restriction: from life extension to life shortening. Aging cell, 9(1), 92-95.
- ↑ de Cabo, R., & Mattson, M. P. (2019). Effects of intermittent fasting on health, aging, and disease. New England Journal of Medicine, 381(26), 2541-2551.
- ↑ Lee, M. B., Hill, C. M., Bitto, A., & Kaeberlein, M. (2021). Antiaging diets: Separating fact from fiction. Science, 374(6570), eabe7365.
- ↑ Song, J., Jiang, G., Zhang, J., Guo, J., Li, Z., Hao, K., ... & Dai, F. (2019). Metformin prolongs lifespan through remodeling the energy distribution strategy in silkworm, Bombyx mori. Aging (Albany NY), 11(1), 240.
- ↑ Novelle, M. G., Ali, A., Diéguez, C., Bernier, M., & de Cabo, R. (2016). Metformin: a hopeful promise in aging research. Cold Spring Harbor perspectives in medicine, 6(3), a025932.
- ↑ 45.0 45.1 45.2 Barzilai, N., Crandall, J. P., Kritchevsky, S. B., & Espeland, M. A. (2016). Metformin as a tool to target aging. Cell metabolism, 23(6), 1060-1065.
- ↑ Campbell, J. M., Bellman, S. M., Stephenson, M. D., & Lisy, K. (2017). Metformin reduces all-cause mortality and diseases of ageing independent of its effect on diabetes control: a systematic review and meta-analysis. Ageing Research Reviews, 40, 31-44.
- ↑ Partridge, L., Fuentealba, M., & Kennedy, B. K. (2020). The quest to slow ageing through drug discovery. Nature Reviews Drug Discovery, 19(8), 513-532.
- ↑ Blagosklonny, M. V. (2021). The goal of geroscience is life extension. Oncotarget, 12(3), 131.
- ↑ Fontana, L., Partridge, L., & Longo, V. D. (2010). Extending healthy life span—from yeast to humans. science, 328(5976), 321-326.
- ↑ 50.0 50.1 Blagosklonny, M. (2019). Rapamycin for longevity: opinion article. Aging, 11(19), 8048-8067. https://doi.org/10.18632/aging.102355
- ↑ Johnson, S. C., Rabinovitch, P. S., & Kaeberlein, M. (2013). mTOR is a key modulator of ageing and age-related disease. Nature, 493(7432), 338-345.
- ↑ Sirolimus - Wikipedia. En.wikipedia.org. (2021). Retrieved 27 May 2021, from https://en.wikipedia.org/wiki/Sirolimus.
- ↑ Blagosklonny, M. (2006). Aging and Immortality: Quasi-Programmed Senescence and Its Pharmacologic Inhibition. Cell Cycle, 5(18), 2087-2102. https://doi.org/10.4161/cc.5.18.3288
- ↑ Johnson, S. C., Martin, G. M., Rabinovitch, P. S., & Kaeberlein, M. (2013). Preserving youth: does rapamycin deliver?. Science translational medicine, 5(211), 211fs40.
- ↑ Johnson, S. C., Martin, G. M., Rabinovitch, P. S., & Kaeberlein, M. (2013). Preserving youth: does rapamycin deliver?. Science translational medicine, 5(211), 211fs40.
- ↑ Kaeberlein, M. (2014). Rapamycin and aging: when, for how long, and how much? Journal of genetics and genomics, 41(9), 459.
- ↑ Participatory Evaluation (of) Aging (With) Rapamycin (for) Longevity Study - Full Text View - ClinicalTrials.gov. Clinicaltrials.gov. (2021). Retrieved 27 May 2021, from https://clinicaltrials.gov/ct2/show/NCT04488601.
- ↑ Ogden, L. E. (2019). Travels through Time. BioScience, 69(11), 860-866.
- ↑ Margolick, J. B., & Ferrucci, L. (2015). Accelerating aging research: how can we measure the rate of biologic aging?. Experimental gerontology, 64, 78-80.
- ↑ 60.0 60.1 Govindaraju, D., Atzmon, G., & Barzilai, N. (2015). Genetics, lifestyle and longevity: lessons from centenarians. Applied & translational genomics, 4, 23-32.
- ↑ Andersen, S. L., Sebastiani, P., Dworkis, D. A., Feldman, L., & Perls, T. T. (2012). Health span approximates life span among many supercentenarians: compression of morbidity at the approximate limit of life span. Journals of Gerontology Series A: Biomedical Sciences and Medical Sciences, 67(4), 395-405.
- ↑ 62.0 62.1 Santesmasses, D., Castro, J. P., Zenin, A. A., Shindyapina, A. V., Gerashchenko, M. V., Zhang, B., ... & Gladyshev, V. N. (2020). COVID‐19 is an emergent disease of aging. Aging cell, 19(10), e13230.
- ↑ Promislow, D. E. (2020). A geroscience perspective on COVID-19 mortality. The Journals of Gerontology: Series A, 75(9), e30-e33.
- ↑ Alberts, S. C., Archie, E. A., Gesquiere, L. R., Altmann, J., Vaupel, J. W., & Christensen, K. (2014). The male-female health-survival paradox: a comparative perspective on sex differences in aging and mortality. In Sociality, hierarchy, health: comparative biodemography: a collection of papers. National Academies Press (US).
- ↑ 65.0 65.1 65.2 Sierra, F. (2020). Geroscience and the Coronavirus Pandemic: The Whack‐a‐Mole Approach is not Enough. Journal of the American Geriatrics Society, 68(5), 951.
- ↑ 66.0 66.1 Barzilai, N., Appleby, J. C., Austad, S. N., Cuervo, A. M., Kaeberlein, M., Gonzalez-Billault, C., ... & Sierra, F. (2020). Geroscience in the Age of COVID-19. Aging and disease, 11(4), 725.
- ↑ https://www.ncbi.nlm.nih.gov/pmc/articles/PMC7288963/
- ↑ O’Keeffe, L. M., Taylor, G., Huxley, R. R., Mitchell, P., Woodward, M., & Peters, S. A. (2018). Smoking as a risk factor for lung cancer in women and men: a systematic review and meta-analysis. BMJ open, 8(10), e021611.
- ↑ Williamson, E. J., Walker, A. J., Bhaskaran, K., Bacon, S., Bates, C., Morton, C. E., ... & Goldacre, B. (2020). Factors associated with COVID-19-related death using OpenSAFELY. Nature, 584(7821), 430-436.
- ↑ Polidori, M. C., Sies, H., Ferrucci, L., & Benzing, T. (2021). COVID-19 mortality as a fingerprint of biological age. Ageing Research Reviews, 101308.
- ↑ Gupta, A., Madhavan, M. V., Sehgal, K., Nair, N., Mahajan, S., Sehrawat, T. S., ... & Landry, D. W. (2020). Extrapulmonary manifestations of COVID-19. Nature medicine, 26(7), 1017-1032.
- ↑ Murray, K. O., Mahoney, S. A., Venkatasubramanian, R., Seals, D. R., & Clayton, Z. S. (2023). Aging, aerobic exercise, and cardiovascular health: Barriers, alternative strategies and future directions. Experimental gerontology, 173, 112105.PMID: 36731386 PMC:10068966 DOI: 10.1016/j.exger.2023.112105
- ↑ Mahmooda, S. S., Levy, D., Vasan, R. S., & Wang, T. J. (2014). The Framingham Heart Study and the epidemiology of cardiovascular diseases: A historical perspective. Lancet, 383(9921), 999-1008.
- ↑ Hajar, R. (2016). Framingham contribution to cardiovascular disease. Heart views: the official journal of the Gulf Heart Association, 17(2), 78.
- ↑ Kaeberlein, M., Rabinovitch, P. S., & Martin, G. M. (2015). Healthy aging: the ultimate preventative medicine. Science, 350(6265), 1191-1193.
- ↑ Olshansky, S. J. (2015). Has the rate of human aging already been modified?. Cold Spring Harbor perspectives in medicine, 5(12), a025965.
- ↑ https://ourworldindata.org/age-structure?source=content_type%3Areact%7Cfirst_level_url%3Aarticle%7Csection%3Amain_content%7Cbutton%3Abody_link
- ↑ Lutz, W., Sanderson, W., & Scherbov, S. (2008). The coming acceleration of global population ageing. Nature, 451(7179), 716-719.
- ↑ 79.0 79.1 79.2 Jin, K., Simpkins, J. W., Ji, X., Leis, M., & Stambler, I. (2015). The critical need to promote research of aging and aging-related diseases to improve health and longevity of the elderly population. Aging and disease, 6(1), 1.
- ↑ Wertz, J., Caspi, A., Ambler, A., Broadbent, J., Hancox, R. J., Harrington, H., ... & Moffitt, T. E. (2021). Association of History of Psychopathology With Accelerated Aging at Midlife. JAMA psychiatry.
- ↑ Bersani, F. S., Mellon, S. H., Reus, V. I., & Wolkowitz, O. M. (2019). Accelerated aging in serious mental disorders. Current opinion in psychiatry, 32(5), 381.
- ↑ Yegorov, Y. E., Poznyak, A. V., Nikiforov, N. G., Sobenin, I. A., & Orekhov, A. N. (2020). The link between chronic stress and accelerated aging. Biomedicines, 8(7), 198.
- ↑ Crimmins, E. M., Kim, J. K., & Seeman, T. E. (2009). Poverty and biological risk: the earlier “aging” of the poor. Journals of Gerontology Series A: Biomedical Sciences and Medical Sciences, 64(2), 286-292.
- ↑ [./Https://clinicalepigeneticsjournal.biomedcentral.com/articles/10.1186/s13148-019-0777-z Wu, X., Huang, Q., Javed, R., Zhong, J., Gao, H., & Liang, H. (2019). Effect of tobacco smoking on the epigenetic age of human respiratory organs. Clinical epigenetics, 11(1), 1-9.]
- ↑ Aung, H. L., Aghvinian, M., Gouse, H., Robbins, R. N., Brew, B. J., Mao, L., & Cysique, L. A. (2020). Is There Any Evidence of Premature, Accentuated and Accelerated Aging Effects on Neurocognition in People Living with HIV? A Systematic Review. AIDS and Behavior, 1-44.
- ↑ Aguayo-Mazzucato, C., Andle, J., Lee Jr, T. B., Midha, A., Talemal, L., Chipashvili, V., ... & Bonner-Weir, S. (2019). Acceleration of β cell aging determines diabetes and senolysis improves disease outcomes. Cell metabolism, 30(1), 129-142.
- ↑ Gensous, N., Bacalini, M. G., Franceschi, C., & Garagnani, P. (2020, July). Down syndrome, accelerated aging and immunosenescence. In Seminars in Immunopathology (pp. 1-11). Springer Berlin Heidelberg.
- ↑ Yamaga, M., Takemoto, M., Shoji, M., Sakamoto, K., Yamamoto, M., Ishikawa, T., ... & Yokote, K. (2017). Werner syndrome: a model for sarcopenia due to accelerated aging. Aging (Albany NY), 9(7), 1738.
- ↑ 89.0 89.1 Guida, J. L., Agurs-Collins, T., Ahles, T. A., Campisi, J., Dale, W., Demark-Wahnefried, W., ... & Ness, K. K. (2020). Strategies to Prevent or Remediate Cancer and Treatment-Related Aging. JNCI: Journal of the National Cancer Institute
- ↑ Kohanski, R. A., Deeks, S. G., Gravekamp, C., Halter, J. B., High, K., Hurria, A., ... & Sierra, F. (2016). Reverse geroscience: how does exposure to early diseases accelerate the age‐related decline in health? Annals of the New York Academy of Sciences, 1386, 30-44
- ↑ https://www.cnbc.com/2019/05/08/techs-next-big-disruption-could-be-delaying-death.html
- ↑ 92.0 92.1 Scott, A. J., Ellison, M., & Sinclair, D. A. (2021). The economic value of targeting aging. Nature Aging, 1(7), 616-623.
- ↑ Crimmins, E. M. (2011). Beltrán-‐Sánchez, H.(2010). Mortality and morbidity trends: Is there compression of morbidity. Journal of Gerontology: Social Sciences, 66B, 1.
- ↑ 94.0 94.1 Keyfitz, N. (1977). What difference would it make if cancer were eradicated? An examination of the Taeuber paradox. Demography, 14(4), 411-418.
- ↑ 95.0 95.1 Mitra, S. (1978). A short note on the Taeuber paradox. Demography, 15(4), 621-623.
- ↑ 96.0 96.1 96.2 Kaeberlein, M. (2017). Translational geroscience: A new paradigm for 21st century medicine. Translational medicine of aging, 1, 1-4.
- ↑ Armstrong, G. L., Conn, L. A., & Pinner, R. W. (1999). Trends in infectious disease mortality in the United States during the 20th century. Jama, 281(1), 61-66.
- ↑ Fauci, A. S. (2001). Infectious diseases: considerations for the 21st century. Clinical Infectious Diseases, 32(5), 675-685.
- ↑ Arias, E., Heron, M. P., & Tejada-Vera, B. (2013). United States life tables eliminating certain causes of death, 1999-2001.
- ↑ Hayflick, L. (2021). The greatest risk factor for the leading cause of death is ignored. Biogerontology, 22(1), 133-141.
- ↑ Prasanna, P. G., Citrin, D. E., Hildesheim, J., Ahmed, M. M., Venkatachalam, S., Riscuta, G., ... & Coleman, C. N. (2021). Therapy-Induced Senescence: Opportunities to Improve Anti-Cancer Therapy. JNCI: Journal of the National Cancer Institute.
- ↑ Hubbard, R. E., O’Mahony, M. S., Savva, G. M., Calver, B. L., & Woodhouse, K. W. (2009). Inflammation and frailty measures in older people. Journal of cellular and molecular medicine, 13(9b), 3103-3109.
- ↑ Leng, S. X., Xue, Q. L., Tian, J., Walston, J. D., & Fried, L. P. (2007). Inflammation and frailty in older women. Journal of the American Geriatrics Society, 55(6), 864-871.
- ↑ Kaeberlein, M. (2019). It is time to embrace 21st-century medicine. public policy & aging report, 29(4), 111-115
- ↑ 105.0 105.1 Chiong, W. (2001). Diagnosing and defining disease. JAMA, 285(1), 89-90.
- ↑ Brown, W. M. (1985). On defining ‘disease’. The Journal of Medicine and Philosophy, 10(4), 311-328.
- ↑ Larsson, L. (2019). Dealing with Death: The Romanticising of Tuberculosis in Three Victorian Novels.
- ↑ Latimer, D. (1990). Erotic susceptibility and tuberculosis: literary images of a pathology. MLN, 105(5), 1016-1031.
- ↑ 109.0 109.1 Rattan, S. I. (2014). Aging is not a disease: implications for intervention. Aging and disease, 5(3), 196.
- ↑ 110.0 110.1 Head, T., Daunert, S., & Goldschmidt-Clermont, P. J. (2017). The aging risk and atherosclerosis: a fresh look at arterial homeostasis. Frontiers in genetics, 8, 216.
- ↑ 111.0 111.1 Anker, S. D., Morley, J. E., & von Haehling, S. (2016). Welcome to the ICD‐10 code for sarcopenia.
- ↑ Calimport, S. R., Bentley, B. L., Stewart, C. E., Pawelec, G., Scuteri, A., Vinciguerra, M., ... & Church, G. (2019). To help aging populations, classify organismal senescence. Science, 366(6465), 576-578.
- ↑ Zhavoronkov, A., & Bhullar, B. (2015). Classifying aging as a disease in the context of ICD-11. Frontiers in genetics, 6, 326.
- ↑ Bulterijs, S., Hull, R. S., Björk, V. C., & Roy, A. G. (2015). It is time to classify biological aging as a disease. Frontiers in genetics, 6, 205.
- ↑ Kirkwood, T. B. (2003). The most pressing problem of our age. Bmj, 326(7402), 1297-1299.
- ↑ Gladyshev, T. V., & Gladyshev, V. N. (2016). A disease or not a disease? Aging as a pathology. Trends in molecular medicine, 22(12), 995-996.
- ↑ Faragher, R. G. (2015). Should we treat aging as a disease? The consequences and dangers of miscategorisation. Frontiers in genetics, 6, 171.